利用者:ProfessorPine/sandbox8
![]() | ハビタブルゾーンの改訳:英語版 Circumstellar habitable zone 14:37, 19 December 2019 (UTC) (oldid=931543450) と 日本語版 ハビタブルゾーン 2020年1月9日 (木) 09:39 (UTC) (oldid=75696764) をマージして改訳作業中。英語版著作権侵害の玉突き事故処理済バージョン。 |

天文学におけるハビタブルゾーン[1][2](英語: Habitable zone、略称: HZ)とは、地球と似た生命が存在できる宇宙空間を指し[1]、日本語では生命居住可能領域[1][3]や生存可能圏[1]、生存可能領域[4]とも呼ばれる。一般的にハビタブルゾーンという用語は惑星系のハビタブルゾーン(英語: Circumstellar habitable zone、略称: CHZ)を指すことが多く、具体的には惑星の表面上に適度な温度、気体の酸素、そして液体の水の3点があることが生命居住の条件となる[5][注 1]。つまり水が蒸発したり凍結しないよう、放射エネルギーを発する恒星(太陽など)から適度な距離に惑星が位置していること、そして受け取ったエネルギー(温度)を逃がさぬよう、惑星に十分な大気圧があって温室効果が得られることがハビタブルゾーンには不可欠となる[6][7]。1953年、米国の天文学者ハーロー・シャプレーによってハビタブルゾーンの概念が初めて提唱されたが[8]、当初からその定義・基準を巡って疑問が呈され続けられており、いまだ発展途上の理論でもある[9]。
さらに、太陽系外でも惑星などの周りを公転する衛星には惑星と同規模のものも多数存在し、これら衛星が太陽系の惑星数を上回る形でハビタブルゾーン内に点在しうることから、惑星ではなく衛星の居住可能性に着目する専門家もいる[10]。また、銀河系のハビタブルゾーン(英語: Galactic habitable zone、略称: GHZ)や[11]、ブラックホールのハビタブルゾーンなど[12][13]、惑星系以外のハビタブルゾーンの理論も提唱されており[14]、本項で併せて解説する。
ハビタブルゾーンは時にゴルディロックスゾーン(英語: Goldilocks zone、略称: GZ)とも呼ばれ[15]、これは童話『3びきのくま』に由来する。少女ゴルディロックは寒暖や大小など極端なものを避け、ちょうどいい中庸を選ぶ性格であることから、ゴルディロックスゾーンという用語は単に生命体が存在しうるだけでなく、進化が起きるのにも適した領域(狭義のハビタブルゾーン)を指す場合もある[2]。つまり、地球上の生物に似た地球外生命体、そして地球外知的生命体が存在する可能性がある宇宙領域である。
こうした理論に基づいて観測が進められ、恒星の周りを公転する惑星がハビタブルゾーン内に存在するケースが多数確認されており、その一部には複数の惑星が公転している惑星系も含まれる[16]。特に地球よりも大きな惑星であるスーパー・アースや巨大ガス惑星は発見が容易なことから、ハビタブルゾーン内で観測されやすい傾向にある。また惑星系以外の研究も進んでおり、2013年11月4日、太陽系外惑星探査望遠鏡ケプラーの観測データに基づく共著論文が『米国科学アカデミー紀要』上で発表された。これによると、銀河系に存在する太陽に似た恒星や赤色矮星の周りを公転し、かつハビタブルゾーン内に位置する地球規模の惑星の数は400億個に上ると推定され[17][18]、これらのうち、太陽に類似の恒星を公転する惑星数は最大で110億個に上ると試算されている[19][18]。また別の研究によれば、地球から最も近い距離にあるとされる太陽系外惑星のプロキシマ・ケンタウリb(地球からケンタウルス座の方向に約4.2光年(約1.3パーセク)先)もハビタブルゾーン内に位置しており、主星である赤色矮星プロキシマ・ケンタウリの周りを公転している[20]。
地球外に液体の水が存在するとの実証結果を受け、今日ではハビタブルゾーンは惑星系の外部に大規模に広がっていると考えられている。地球を含む太陽系では、岩石圏(リソスフェア、いわゆる岩盤プレート)やその下層にあたるアセノスフェア内に大量の水が存在する。この事実から宇宙生物学では、恒星からの放射エネルギーを必要としない地下生物圏の概念が通説となっている[21]。放射エネルギーの代替手段としては潮汐加熱(ちょうせきかねつ)[22][23]や放射性崩壊[24]などがあり、また大気以外の理由で気圧が加圧されたりすれば、自由浮遊惑星や太陽系外衛星であっても液体の水が存在する可能性がある[25]。液体の水は何らかの化合物を溶かすことで、沸点・凝固点や蒸気圧が変化することから(これを「束一的性質」と呼ぶ)、例えば地球上の海水中に含まれる塩化ナトリウム、火星の赤道上の塩化物や硫酸塩[26]、そしてアンモニア[27]などの溶液であれば、水が液体の形を維持する可能性が高まる。さらには、炭素や水を必要としない生化学 (alternative biochemistries) の仮説に基づくと、水以外の溶媒が液体の状態で存在さえすれば、水なしでも地球外生命体が生存しうる。この説に立脚すると、ハビタブルゾーンの範囲は従来よりもより広範となる[2][28]。
歴史[編集]
液体の水が存在しうる恒星からの距離範囲を推測する試みは、古くは1687年のアイザック・ニュートン著『自然哲学の数学的諸原理』(第3編「世界体系」第1章 COROL 4)にも見られる[29][疑問点 ]。その後、「ハビタブルゾーン」の概念はドイツの生理学・医学者フベルトゥス・シュトルークホルトによって1953年に初めて示され[要検証 ]、その著書 "The Green and the Red Planet: A Physiological Study of the Possibility of Life on Mars" 内で「エコスフィア」(英語: Ecosphere)という造語を用いて、生命体が出現する可能性がある様々な「ゾーン」について言及している[30][31]。同年にアメリカの天文学者ハーロー・シャプレーは "Liquid Water Belt theory"(仮訳: 液体の水帯域の理論)を提唱し、これが現在のハビタブルゾーン理論の原形とみなされている[32]これは同じ理論を科学的にさらに詳しく述べたものである[要検証 ]。両者の研究は生命体にとっての液体の水の重要性を強調するものである[33]。シャプレーとシュトルークホルトから遅れること1959年、"Habitable zone" という表現を初めて用いたのが、中国出身で米国籍の天体物理学者黄授書(Su-Shu Huang、簡体字: 黄授书; 繁体字: 黃授書)である。黄の定義によるとハビタブルゾーンとは、恒星の周辺において十分な量の液体の水が存在しうる惑星の領域を指し、惑星の居住性と地球外生命体の文脈において初めてハビタブルゾーンの理論を提唱したのが黄である[34][35]。翌1960年、重力の不安定な多重連星系内では、地球外生命体が存在しうるハビタブルゾーンの可能性は低いと黄は論じているその不安定な重力の影響により珍しいものになるであろうと主張している[訳語疑問点][36]。
続く1964年、米国ランド研究所のステファン・H・ドール著『Habitable Planets for Man』(仮訳: 人類居住可能な惑星)によって、ハビタブルゾーンの理論はさらなる進展を遂げる。本書では惑星系のハビタブルゾーンの概念のほか、惑星の居住可能性の決定諸要因が論じられ、銀河系のハビタブルゾーン内に位置する惑星の数は推定で約6億個にのぼるとの結論に達している[37]。同時期には、三大SF作家としても知られる生化学者のアイザック・アシモフが、宇宙移民に関して多角的に研究し、ハビタブルゾーンの概念を世間一般に広めた[38]。「ゴルディロックスゾーン」の用語が登場したのは1970年代のことであり、この用語は特に液体の水が存在するのに「ちょうど良い」温度になっている恒星の周りの領域を指している[39]。1993年、エイムズ研究センター (NASA附属機関) の天文学者ジェームズ・キャスティングが、現在主にハビタブルゾーンとして知られている領域をより正確に識別するために「惑星系のハビタブルゾーン(Circumstellr habitable zone)」の用語を用いた[34]。また、太陽系外惑星のハビタブルゾーンの詳細なモデルを初めて提唱したのもキャスティングである[34][40]。
2000年、古生物学者ピーター・ワードと天文学者のドナルド・E・ブラウンリー[訳語疑問点]が銀河系のハビタブルゾーンを提唱したことで、ハビタブルゾーンの研究はさらに前進し、この共同研究体制には後に天文学者のギレルモ・ゴンザレスも参画している[41][42]。銀河系のハビタブルゾーンとは、銀河核に十分近いため重元素が豊富に存在していることから[訳語疑問点]、生命体が出現する可能性が最も高い領域と定義される。ただし、銀河核に近づきすぎると強力な放射線と重力の影響を受けることから、恒星系や惑星の軌道は高い確率で乱され、生命体の出現は困難だと考えられている[41]。
ワードらの共同研究以降も、宇宙生物学の領域においてハビタブルゾーンの概念・定義をより広く捉える提唱が相次いでいる。従来の定義では液体の水がハビタブルゾーンには不可欠とされていたが、二水素や硫酸、二窒素、ホルムアミド、メタンといった水以外の溶媒を生命存立要件に加える説や、炭素や水を必要としない生化学 (alternative biochemistries) の立場から未知の生命体の存在を支持する説もある[28][疑問点 ]。2013年には、ハビタブルエッジ(英語: Habitable edge)と呼ばれる惑星のハビタブルゾーン(境界線)が提唱されたことにより、ハビタブルゾーン理論はさらに展開した。ハビタブルエッジとは、自然に形成された衛星の軌道が乱されない領域、かつ惑星からの潮汐加熱で表面の液体の水が沸騰せず安定している領域を示す[14]。なお、潮汐加熱を地球(惑星)と月(衛星)で説明すると、月からの引力によって地球上の海洋で潮が満ち引きする原理に関係している。このような体感からイメージできるように、必ずしも天体の表面は安定しておらず、惑星と衛星はその引力の違いによって球体からややずれたラグビーボール状に変形する(これを潮汐作用と呼ぶ)。この変形にさらに公転運動が加わると、(恒星である太陽からの放射エネルギーがなくとも)衛星の中心部で熱を発することから、潮汐加熱と呼ばれている[43][44][疑問点 ]。
It has been noted that the current term of 'circumstellar habitable zone' poses confusion as the name suggests that planets within this region will possess a habitable environment.[34][35] However, surface conditions are dependent on a host of different individual properties of that planet.[34][35] This misunderstanding is reflected in excited reports of 'habitable planets'.[36][37][38] Since it is completely unknown whether conditions on these distant CHZ worlds could host life, different terminology is needed.[35][37][39][40] ←★これが英語版にあるが、日本語版では未訳。たしかに歴史節の最後にこの段落を入れるのはなんだか違和感満載。。。出典の中身をチェックして、他の節で再利用できるか要検討。
ハビタブルゾーンの範囲[編集]
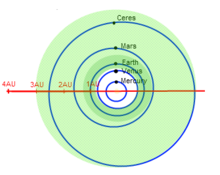
ある天体がその主星のハビタブルゾーン内に位置しているかどうかは、惑星の軌道半径(衛星の場合は主惑星の軌道)、天体自身の質量、および主星の放射束に依存する[46]。すなわちこれを地球に当てはめて簡略化すれば、太陽からの距離、地球の重さ、および陽当たりの強さの3要素によって、地球はハビタブルゾーン内に位置すると定められる。これら3点は、天体の表面温度を左右する。太陽から遠ざかるほど、その天体に届く太陽の放射エネルギーは減り、天体の表面温度は下がる。しかし天体の質量が大きければ、それに比例して重力が働くことから、大気の厚みが増す。この大気が毛布のような保温の役割を果たして温室効果をもたらすことから、たとえ太陽から遠くとも、質量が大きければ表面温度が高まる。地球であれば、反射率を勘案した上で太陽から届く温度(平衡温度)は摂氏マイナス18度だが、実際の地表の平均温度はプラス15度であり、この差分33度は温室効果によってもたらされている[注 2]。一方、金星は分厚い大気をまとっていることから反射率が高く(つまり太陽光が遮られて地表に届きづらく)、地球よりも金星の方が太陽に近いにもかかわらず、平衡温度はマイナス29度と地球よりも低い[注 3]。しかしながら、この大気の厚みによって温室効果が増すため、最終的な金星の表面温度はプラス400度に達し、液体の水は存在しえない[47][疑問点 ]。
したがって、ハビタブルゾーンの範囲を決定する天体の質量は、その上限・下限の幅が広い。また、地球を凌駕するほど分厚い大気と強力な磁場をまとったスーパー・アースが発見されていることから、ハビタブルゾーンの範囲は広範に渡っている。そこで今日では、惑星系のハビタブルゾーンを、地球や金星など比較的低質量の惑星の表面で液体の水が存在できる領域と、より高い温室効果を持つスーパー・アースの表面上で液体の水が存在するのに適した温度になる "Extended habitable zone"(仮訳: 拡張ハビタブルゾーン)と呼ばれるより広範囲の領域に二分して捉えられている[46]。
★この1段落はまだぎこちないので、後で追加改訳する★
ハビタブルゾーンの内縁(恒星に最も近いボーダーライン)は、温室効果によって天体表面の水が蒸発してしまう[9][注 4]。この水蒸気が、毛布のような保温の役割を果たしてさらなる温室効果を発生させ、天体表面の温度がまるで暴走するように上昇する暴走温室効果の状態に陥る[50]。さらにこの水蒸気は、光解離によって分子そのものが分解し、水素として宇宙空間へと放出される[4][51][49]。そのため、一般的にハビタブルゾーンの内縁境界の条件は「暴走温室条件」と呼ばれ、暴走温室効果(もしくはそれより少し効果が弱い湿潤温室効果)が発生してしまう惑星からの射出限界と等しい恒星放射を受ける領域とされる[4][51]。一方で、ハビタブルゾーンの外縁付近では、大気に多くの二酸化炭素が存在していても惑星表面を水の融点よりも高温に保つことができないほど主星からは離れている[9]。外縁境界の条件は「全球凍結条件」と呼ばれ[51]、惑星が全球凍結にならない最低限の恒星放射を受ける領域と定義されている[52][34][4]。
太陽系での推定値[編集]
今日では、太陽系内のハビタブルゾーンは0.38–10.0 auの範囲に位置すると推定されているが[53][54][55][56]、様々な原因によりこの推定値を導き出すのは困難であった。惑星クラスの質量を持ち、かつハビタブルゾーンの推定範囲内もしくはそれに近い軌道を周回している天体は多数存在する。これらの天体には十分な太陽光が降り注いでいることから、表面温度は水の融点を超えるものの、それぞれの大気条件は大きく異なっている。例えば金星の遠日点[注 5]は、ハビタブルゾーンの内縁付近に位置している。金星表面の大気圧は、液体の水を保持するのには十分だが、強い温室効果により表面温度は462 ℃(864 ℉)にまで上昇しており、水は水蒸気でしか存在することができない[58]。ただし金星の上空50 km付近は、太陽系の中では最も地球の環境に近いとされており、50 kmの1気圧地点では75 ℃、55 kmの0.5気圧地点では27 ℃で液体の水が存在できる温度になっている[59]。
月[60]や火星[61]、そして多数の小惑星もまた、ハビタブルゾーンの推定範囲内に位置している。しかしながら火星の場合、水が液体の状態で存在しうる大気圧と温度の条件を満たせるのは、表面全体の30%未満を占める低地のみであり、しかも液体状態を保持できるのは短期間に限られている(あくまで水が存在したと仮定した場合)[62]。例えばヘラス盆地では、年間70火星日の間は大気圧が1,115 Paに達し、温度が0 ℃を超えることがある[62]。火星は乾いた惑星だと考えられてきたが、2011年に火星の斜面に暗い筋模様(通称: RSL)が幾多も発見されたことから、液体の水の存在が示唆されたこともあった[63][64][65][66]。ところが2017年には、この筋模様は液体の水ではなく、砂が斜面を流れた跡の可能性が高いとして、アメリカ地質調査所の宇宙地質学科学センター (Astrogeology Science Center) 研究チームは結論付けている[67]。
ハビタブルゾーン内を公転している天体は、彗星を含め複数存在するが、その中でも準惑星のケレスは唯一惑星クラスの質量を持つ。しかし質量が小さいことに加え、太陽風による水分蒸発と大気の減少の影響を強く受けることから、これらの天体は表面上に液体の水を保持することができない。にも関わらず、かつて金星[68]や火星[69][70]、ベスタ[71]、ケレス[72][73]の表面には、液体の水が存在していた痕跡が高い確率で確認されており、惑星における水の存在は、地球に限った特徴ではなくより普遍性があると今日では考えられている。かつては惑星の軌道は現在とは異なり、表面重力によって十分な大気が数十億年の間存在した時代があったことから、液体の水も存在したと推定されている[要出典]。
拡張ハビタブルゾーンの概念に基づくと、惑星クラスの質量を持ち、かつ十分な放射強制力を持つ大気に包まれた天体は、太陽から遠く離れていても水を液体の状態で保持できる[要出典]。放射強制力とは、地球に入ってくるエネルギー(太陽から放射されるエネルギーなど)と、地球から出ていくエネルギー(地球放射)の熱収支バランスであり、正の値は保温に、負の値は冷却につながる[74][75]。大気中に大量の温室効果ガスが含まれている天体や、地球よりも質量が大きく、表面圧力が最大100 kbarに達するような岩石惑星(スーパー・アースクラスの質量)であれば、液体の水を有することができる。しかし、この条件を満たす天体は太陽系で発見されていない。また、太陽系外惑星の大気特性は研究途上である。ハビタブルゾーン内に天体が存在するというだけでは、実際に液体の水が存在しうるか断定できず、誘導アルベド(Induced albedo)や反温室効果、その他の熱源といった諸条件によって最終的な表面温度が決定される[要出典]。
For reference, the average distance from the Sun of some major bodies within the various estimates of the habitable zone are: Mercury, 0.39 AU; Venus, 0.72 AU; Earth, 1.00 AU; Mars, 1.52 AU; Vesta, 2.36 AU; Ceres, 2.77 AU; Jupiter, 5.20 AU; Saturn, 9.58 AU.
★以下の表内の訳は後でチェックする★
内縁距離 (au) |
外縁距離 (au) |
発表者(発表年) | 注釈 |
---|---|---|---|
0.725 | 1.24 | Dole(1964)[37] | 光学的に薄い大気と固定アルベドを使用して計算された値。金星の遠日点付近に内縁が位置する。 |
1.385–1.398 | Budyko(1969)[76] | 地球が経験するであろう全球規模の凍結の時代を決定するためのアイスアルベドフィードバックモデルの研究に基づいている。この推定は1969年のSellersの研究[77]や1975年のNorthの研究[78]でも支持されている。 | |
0.88–0.912 | RasoolとDe Bergh(1970)[79] | 金星の大気の研究に基づいて、RasoolとDe Berghはこの距離が地球上で安定した海が存在できるであろう最も太陽に近い距離であると結論付けている。 | |
0.95 | 1.01 | Hartら(1979)[80] | 地球の大気組成と地表温度のコンピューターモデリングとシミュレーションに基づいている。この推定は、その後にしばしば出版物で引用されてきた。 |
3.0 | Fogg(1992)[45] | 炭素循環を用いてハビタブルゾーンの外縁距離を推定した。 | |
0.95 | 1.37 | Kastingら(1993)[34] | 今日使用されている最も一般的なハビタブルゾーンの実用的定義を確立した。二酸化炭素と水が地球にとって重要な温室効果ガスであると仮定し、炭酸塩-ケイ酸塩循環(Carbonate-silicate cycle)によりハビタブルゾーンは広いものになっていると主張している。雲のアルベドによるの冷却効果にも注目している。左に記載しているのは控えめな制限を与えた推定で、楽観的な推定に基づくとその範囲は0.84–1.67 auとなる。 |
2.0 | Spiegelら(2010)[81] | 大きい軌道傾斜と離心率を組み合わせると、この距離までなら周期的に液体の水が存在できることが提案された。 | |
0.75 | Abeら(2011)[82] | 地球のような水が多い惑星よりも主星に近く、極付近にのみ水が存在し大部分が陸地を占めている「砂漠惑星(Desert planet)」が存在する可能性を示した。 | |
10 | PierrehumbertとGaidos(2011)[83] | 原始惑星系円盤から気圧数十から数千 barの水素を蓄積することができる岩石惑星は、太陽から10 auも離れた領域でも居住可能になる可能性を示した。 | |
0.77–0.87 | 1.02–1.18 | Vladiloら(2013)[84] | 必要な大気圧の下限を15 mbarとした時、ハビタブルゾーンの内縁はさらに太陽に近く、外縁はさらに遠くなることを示した。 |
0.99 | 1.70 | Kopparapuら(2013)[52][85] | Kastingら(1993)の推定値を修正したもの。更新された湿潤温室効果と水分損失のアルゴリズムを用いて公式化している。この測定によると、地球はハビタブルゾーンの内縁に位置しており、湿潤温室効果が起きる距離の限界に近いがわずかにその外側に位置する。Kastingら(1993)と同じように、 これは温度が60 ℃に達する「水損失(湿潤温室効果)」の限界であるハビタブルゾーンの内縁に位置し、十分高度が高い領域に対流圏があり、大気が完全に水蒸気で飽和している地球のような惑星に適用される。成層圏が湿ると水蒸気光分解により水素が宇宙空間に放出される。この時点では、雲のフィードバックによる冷却は、さらに強い温暖化の効果により著しくは強くならない。「最大温室効果(Maximum greenhouse)」の限界であるハビタブルゾーンの外縁では、二酸化炭素が支配的な気圧約8 barの大気が最も強い温室効果を生み出し、二酸化炭素がさらに増加しても大気圏外で凍結するのを防ぐために十分な温室効果は発生しないとされている。楽観的な推定では範囲は0.97–1.70 auとなっている。この楽観的な推定では、二酸化炭素の雲による放射温暖化の可能性は考慮されていない。 |
0.38 | Zsomら(2013)[53] | 惑星の大気組成、圧力および相対湿度などの考えられる様々な組み合わせに基づいて推定されている。 | |
0.95 | Leconteら(2013)[86] | 3Dモデルを用いて、Leconteらは太陽系のハビタブルゾーンの内縁を0.95 auとした。 | |
0.95 | 2.4 | RamirezとKaltenegger(2017)[55] | 火山性水素の大気濃度を50%と仮定したときの古典的な二酸化炭素と水蒸気のハビタブルゾーンの拡大[34]を示した。 |
太陽系外での推定値[編集]

太陽系のハビタブルゾーン推定モデルは他の惑星系にも援用されており、その際には恒星の放射束と逆2乗の法則が用いられている。太陽系のハビタブルゾーンの中間地点は太陽から1.34 auの距離に位置するが[52]、例えば太陽の0.25倍の光度を持つ恒星の場合、恒星からハビタブルゾーンの中間までの距離は太陽系のハビタブルゾーンの倍、すなわち0.5倍となり、恒星からは0.67 au離れていることになる。しかし、恒星はそれぞれ異なる特性を持つことなどを勘案すると、ハビタブルゾーンの概念の太陽系外への適用は、より複雑化する[要出典]。
スペクトル分類と恒星系の特性[編集]
★改訳作業の続き、ここから★
一部の科学者たちは、惑星系のハビタブルゾーンの概念は実際にはある種の恒星やそのスペクトル分類に限定されるものだと主張している。例えば連星では、三重連星系の場合における固有の軌道安定性の懸念も加えて、単一星の場合とは異なるハビタブルゾーンを持つ[87]。もし太陽系がそのような連星系であった場合、結果として得られる外縁までの距離は2.4 auにまで及んでいたかもしれない[88][89]。
Some scientists argue that the concept of a circumstellar habitable zone is actually limited to stars in certain types of systems or of certain spectral types. Binary systems, for example, have circumstellar habitable zones that differ from those of single-star planetary systems, in addition to the orbital stability concerns inherent with a three-body configuration.[73] If the Solar System were such a binary system, the outer limits of the resulting circumstellar habitable zone could extend as far as 2.4 AU.[74][75]
恒星のスペクトル分類について、ハンガリーの天文学者Zoltán Balogは、強い紫外線を放射しているO型主系列星の周辺では惑星は形成されないことを示している[90]。また、紫外線の放射について調査したAndrea Buccinoらの研究チームは、調査を行った恒星(太陽も含む)のうち40%のみが、ハビタブルゾーンと適度な紫外線を受ける領域とが重なることを発見している[91]。一方で、太陽より小さな恒星には居住性に明らかな障害が見られる。例えば天文学者Michael Hartは、スペクトル分類K0型もしくはそれより明るい主系列星のみがハビタブルゾーンを持てることを提案しており、現在ではこの主張は赤色矮星の周りを公転する惑星における潮汐固定半径の概念に発展している。赤色矮星系はこの半径とハビタブルゾーンが一致し、主星との潮汐力による加熱(潮汐加熱)で引き起こされた火山活動によって、高温で生命の存在に適さない金星のような惑星が形成されてしまう可能性が示唆されている[92]。
With regard to spectral types, Zoltán Balog proposes that O-type stars cannot form planets due to the photoevaporation caused by their strong ultraviolet emissions.[76] Studying ultraviolet emissions, Andrea Buccino found that only 40% of stars studied (including the Sun) had overlapping liquid water and ultraviolet habitable zones.[77] Stars smaller than the Sun, on the other hand, have distinct impediments to habitability. For example, Michael Hart proposed that only main-sequence stars of spectral class K0 or brighter could offer habitable zones, an idea which has evolved in modern times into the concept of a tidal locking radius for red dwarfs. Within this radius, which is coincidental with the red-dwarf habitable zone, it has been suggested that the volcanism caused by tidal heating could cause a "tidal Venus" planet with high temperatures and no hospitable environment to life.[78]
他の天文学者の中には、ハビタブルゾーンはより一般的なもので、温度が低い恒星を公転している惑星であっても液体の水は存在できると主張している者もいる。2013年に発表された気候モデリングでは、潮汐固定を起こしている惑星であっても、赤色矮星が惑星を一定の温度に保たせる可能性が示された[93]。天文学教授のEric Agolは、白色矮星の周辺を公転する惑星であっても惑星移動を通じて比較的短期間の間、ハビタブルゾーンを維持できると主張している[94]。また中には、褐色矮星の周りにも準安定的にハビタブルゾーンが同様に存在できると主張している者もいる[92]。また、恒星の進化の過程において前主系列星の段階、特にその恒星が赤色矮星である場合、恒星系の外縁部にハビタブルゾーンが存在する可能性があり、潜在的には約10億年に渡って持続されるとされている[95]。
Others maintain that circumstellar habitable zones are more common, and that it is indeed possible for water to exist on planets orbiting cooler stars. Climate modelling from 2013 supports the idea that red dwarf stars can support planets with relatively constant temperatures over their surfaces in spite of tidal locking.[79] Astronomy professor Eric Agol [de] argues that even white dwarfs may support a relatively brief habitable zone through planetary migration.[80] At the same time, others have written in similar support of semi-stable, temporary habitable zones around brown dwarfs.[78] Also, a habitable zone in the outer parts of stellar systems may exist during the pre-main-sequence phase of stellar evolution, especially around M-dwarfs, potentially lasting for billion-year timescales.[81]
恒星の進化との関係[編集]

ハビタブルゾーンは主星の進化に伴って時間を追うごとに変化していく。例えば、1000万年程度しか主系列星の段階を維持しないとされる[96]高温のO型星の場合、生命の進化が追い付かないほど急速に変化するハビタブルゾーンを持つとされている。一方で赤色矮星は、何千億年にも渡って主系列星の段階を維持するため、生命が発達して進化を起こすのに十分な時間がある惑星を持つ事ができる[97][98]。しかし主星が主系列星の段階にあっても、そのエネルギー放射は時間が経過するごとに増加していき、ハビタブルゾーンを遠方に追いやってしまう。例えば太陽も、太古代の明るさは現在の75%しかなかったとされており[99]、将来的に太陽が赤色巨星に進化する前であっても継続的に増加するエネルギー放射により、地球をハビタブルゾーンの内側に追いやるとされている[100]。この明るさの増加に対処するために「継続的なハビタブルゾーン(Continuously habitable zone)」の概念が導入されている。これは名称の通り、恒星の周辺で絶え間なく居住することができる領域のことを指しており、そこでは惑星クラスの質量を持つ天体は、与えられた期間の間液体の水を維持することができる。一般的なハビタブルゾーンと同様に、「継続的なハビタブルゾーン」も保守的な領域と拡張された領域とに分けることができる[100]。
赤色矮星では、わずか数分で恒星全体の明るさが元の2倍にまで明るくなるほどの大規模なフレアや[101]、表面積の20%を占める巨大な恒星黒点が発生することがあり[102]、ハビタブルゾーン内にある惑星の大気と水が失われてしまう可能性がある[103]。しかし、より大きな恒星と同様に進化の過程においてその性質や放射束エネルギーを変えるので[104]、形成から約12億年が経過するまでは赤色矮星は、その惑星上で生命の発達させるのには十分に一定の状態を保つとされている[103][105]。
恒星が赤色巨星にまで進化すると、そのハビタブルゾーンの領域は主系列星の段階から劇的に変化する[106]。例えば太陽の場合、赤色巨星に進化すると現在はハビタブルゾーンに位置している地球も太陽に飲み込まれると予想されている[107][108]。しかしながら、赤色巨星が水平分枝に一旦進化すると、再び恒星全体の均衡が保たれるようになり、太陽の場合だと7–22 au離れた領域が新たなハビタブルゾーンとして維持されるとされている[109]。この段階になると、土星の衛星であるタイタンが現在の地球と似通った温度になるだろう[110]。この均衡状態が約10億年の間続き、なおかつ地球上の生命が太陽系の形成から遅くとも7億年後までに出現しているということを考えると、赤色巨星の周辺のハビタブルゾーン内を公転している惑星クラスの質量を持つ天体であっても生命が発達できる可能性がある[109]。しかし、光合成のような重要な生命過程は大気に二酸化炭素を含む惑星でのみ起こり得るが、そのようなヘリウムを燃焼して均衡を保っている恒星の周囲を公転する惑星ではその多くが恒星に吸収されてしまう[111]。さらに、2016年にRamirezとKalteneggerが示したように[108]、その強い恒星風は惑星の大気を完全に吹き飛ばし、よりそのような惑星を居住不可能にするだろう。したがって、太陽が赤色巨星になった後でさえタイタンは居住可能にならないとされている。ただし、生命の存在が検出されるために恒星進化のこの段階で生命が出現する必要は無い。恒星が赤色巨星になり、ハビタブルゾーンが外側に広がると表面の氷が溶けて、赤色巨星になる前に繁殖していたかもしれない生命の兆候を見出すことができる一時的な大気が形成されるとされている[108]。
Circumstellar habitable zones change over time with stellar evolution. For example, hot O-type stars, which may remain on the main sequence for fewer than 10 million years,[82] would have rapidly changing habitable zones not conducive to the development of life. Red dwarf stars, on the other hand, which can live for hundreds of billions of years on the main sequence, would have planets with ample time for life to develop and evolve.[83][84] Even while stars are on the main sequence, though, their energy output steadily increases, pushing their habitable zones farther out; our Sun, for example, was 75% as bright in the Archaean as it is now,[85] and in the future, continued increases in energy output will put Earth outside the Sun's habitable zone, even before it reaches the red giant phase.[86] In order to deal with this increase in luminosity, the concept of a continuously habitable zone has been introduced. As the name suggests, the continuously habitable zone is a region around a star in which planetary-mass bodies can sustain liquid water for a given period of time. Like the general circumstellar habitable zone, the continuously habitable zone of a star is divided into a conservative and extended region.[86]
In red dwarf systems, gigantic stellar flares which could double a star's brightness in minutes[87] and huge starspots which can cover 20% of the star's surface area,[88] have the potential to strip an otherwise habitable planet of its atmosphere and water.[89] As with more massive stars, though, stellar evolution changes their nature and energy flux,[90] so by about 1.2 billion years of age, red dwarfs generally become sufficiently constant to allow for the development of life.[89][91]
Once a star has evolved sufficiently to become a red giant, its circumstellar habitable zone will change dramatically from its main-sequence size.[92] For example, the Sun is expected to engulf the previously-habitable Earth as a red giant.[93][94] However, once a red giant star reaches the horizontal branch, it achieves a new equilibrium and can sustain a new circumstellar habitable zone, which in the case of the Sun would range from 7 to 22 AU.[95] At such stage, Saturn's moon Titan would likely be habitable in Earth's temperature sense.[96] Given that this new equilibrium lasts for about 1 Gyr, and because life on Earth emerged by 0.7 Gyr from the formation of the Solar System at latest, life could conceivably develop on planetary mass objects in the habitable zone of red giants.[95] However, around such a helium-burning star, important life processes like photosynthesis could only happen around planets where the atmosphere has carbon dioxide, as by the time a solar-mass star becomes a red giant, planetary-mass bodies would have already absorbed much of their free carbon dioxide.[97] Moreover, as Ramirez and Kaltenegger (2016)[94] showed, intense stellar winds would completely remove the atmospheres of such smaller planetary bodies, rendering them uninhabitable anyway. Thus, Titan would not be habitable even after the Sun becomes a red giant.[94] Nevertheless, life need not originate during this stage of stellar evolution for it to be detected. Once the star becomes a red giant, and the habitable zone extends outward, the icy surface would melt, forming a temporary atmosphere that can be searched for signs of life that may have been thriving before the start of the red giant stage.[94]
砂漠惑星[編集]
大気条件は惑星の熱を保持する能力に影響を与えるので、ハビタブルゾーンの位置はそれぞれのタイプの惑星にとっても特有なものになっている。含まれる水の量が非常に少ない砂漠惑星(英語: Desert planet、Dry planet)は、大気中の水蒸気も少なくなるので、温室効果が減少する。これは砂漠惑星では、太陽から地球までの距離よりも恒星に近い領域で水のオアシスを維持できることを意味している。水が不足しているということはまた、熱を宇宙空間に反射するための氷が少なくなることを意味しているので、砂漠惑星にとってのハビタブルゾーンの外縁はより遠い位置になる[112][113]。
A planet's atmospheric conditions influence its ability to retain heat, so that the location of the habitable zone is also specific to each type of planet: desert planets (also known as dry planets), with very little water, will have less water vapor in the atmosphere than Earth and so have a reduced greenhouse effect, meaning that a desert planet could maintain oases of water closer to its star than Earth is to the Sun. The lack of water also means there is less ice to reflect heat into space, so the outer edge of desert-planet habitable zones is further out.[98][99]
その他の考慮事項[編集]
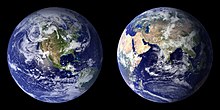
恒星系の中に水の供給源が無ければ、惑星は炭素を基盤とする声明を形成するための重要な要素である水圏を持つことが出来ない。地球の水の起源はまだ完全には解明されていない。考えられる可能性としては、氷で出来た天体との衝突、ガス放出、石灰化、リソスフェアからの含水鉱物の漏出、光分解による結果などが挙げられる[114][115]。太陽系外の惑星系では、凍結線より遠い領域から氷でできた天体がハビタブルゾーン内に移動して水深が数百 kmにも及ぶ[116]、GJ 1214 b[117][118]やケプラー22bのような海洋惑星が形成される可能性がある[119]。
表面に液体の水を維持するには十分に厚い大気も必要となる。地球の大気の起源としては現在、内部からのガス放出や天体衝突によるガスの減少、外部からのガス吸収(Ingassing)によるものと結論付けられている[120]。大気は、生物地球化学的循環および大気放出の緩和に類似したプロセスを通じて維持されると考えられている[121]。イタリアの天文学者Giovanni Vladiloらによる2013年の研究では、惑星の大気圧が大きくなるにつれて恒星周辺のハビタブルゾーンの領域が大きくなることが示された[84]。また、約15 mbar以下の大気圧では、圧力または温度のわずかな変化でも水が液体として存在することが不可能になる可能性があるため、居住性を維持できないことが判明した[84]。
ハビタブルゾーンの範囲の定義は、伝統的に(地球上に存在している)水蒸気と二酸化炭素が最も重要な温室効果ガスと仮定して決定されているが[14]、Ramses RamirezとLisa Kalteneggerによって導かれた研究では、驚異的な水素の火山性ガス放出も水蒸気や二酸化炭素と同じように温室効果ガスに含まれるとすると、ハビタブルゾーンの範囲が非常に広くなることを示された[55]。その場合、太陽系のハビタブルゾーンの外縁は2.4 auにまで遠ざかる。 初期のRay PierrehumbertとEric Gaidosによる研究では、二酸化炭素と水という概念を完全に排除して、若い惑星は原始惑星系円盤から気圧数十から数百 barの水素を蓄積し、十分な温室効果を起こせると主張した[83]。この場合、太陽系のハビタブルゾーンの境界は10 auにまで広がる。しかしこの場合だと、水素は火山活動によって継続的に供給されるわけではないので、数百万から数千万年の間に失われてしまう。
A planet cannot have a hydrosphere—a key ingredient for the formation of carbon-based life—unless there is a source for water within its stellar system. The origin of water on Earth is still not completely understood; possible sources include the result of impacts with icy bodies, outgassing, mineralization, leakage from hydrous minerals from the lithosphere, and photolysis.[100][101] For an extrasolar system, an icy body from beyond the frost line could migrate into the habitable zone of its star, creating an ocean planet with seas hundreds of kilometers deep[102] such as GJ 1214 b[103][104] or Kepler-22b may be.[105]
Maintenance of liquid surface water also requires a sufficiently thick atmosphere. Possible origins of terrestrial atmospheres are currently theorised to outgassing, impact degassing and ingassing.[106] Atmospheres are thought to be maintained through similar processes along with biogeochemical cycles and the mitigation of atmospheric escape.[107] In a 2013 study led by Italian astronomer Giovanni Vladilo, it was shown that the size of the circumstellar habitable zone increased with greater atmospheric pressure.[70] Below an atmospheric pressure of about 15 millibars, it was found that habitability could not be maintained[70] because even a small shift in pressure or temperature could render water unable to form as a liquid.[108]
Although traditional definitions of the habitable zone assume that carbon dioxide and water vapor are the most important greenhouse gases (as they are on the Earth),[25] a study[45] led by Ramses Ramirez and co-author Lisa Kaltenegger has shown that the size of the habitable zone is greatly increased if prodigious volcanic outgassing of hydrogen is also included along with the carbon dioxide and water vapor. The outer edge in our solar system would extend out as far as 2.4 AU in that case. Similar increases in the size of the habitable zone were computed for other stellar systems. An earlier study by Ray Pierrehumbert and Eric Gaidos [44] had eliminated the CO2-H2O concept entirely, arguing that young planets could accrete many tens to hundreds of bars of hydrogen from the protoplanetary disc, providing enough of a greenhouse effect to extend the solar system outer edge to 10 AU. In this case, though, the hydrogen is not continuously replenished by volcanism, and is lost within millions to tens-of-millions of years.
赤色矮星のハビタブルゾーン内を公転する惑星の場合、主星に非常に近い位置にあると潮汐固定が引き起こされ、居住性において重要な要素となる。潮汐固定されている惑星は恒星日が公転周期と同じくらい長くなり、片面を常に主星に向け、もう片面を常にその反対側に向けることになる。過去には、常に主星を向いている面では極端に温度が高くなり、その反対側では極端に温度が低くなると考えられていた。しかし2013年に発表された3次元気候モデルでは、主星に向けている面の広範囲で雲が発生することができ、ボンドアルベドが増加して両側の温度差が大幅に減少することが示された[93]。
惑星ほどの質量を持つ衛星にも居住できる可能性がある。しかし、これらの衛星にはさらに多くのパラメーター条件を満たすことが必要で、特に主惑星が主星のハビタブルゾーン内を公転していることが必要となる[14]。さらに具体的には、このような衛星はイオのような潮汐加熱によって火山で満たされた天体にならないよう、主惑星から十分に離れなければいけないが[14]、外部へ放り出されないように主惑星のヒル半径よりは内側に留まる必要もある[122]。また、太陽の20%以下の質量しか持たない赤色矮星を公転する巨大惑星は居住可能な衛星を持つ事は出来ない。そのような惑星系で衛星が軌道を維持するには、強い潮汐加熱が起きるのに十分なほど主惑星に近い軌道にある必要があり、居住性は見込めなくなる[14]。

高い軌道離心率で恒星を周回する惑星は、公転周期の一部がハビタブルゾーン内を通過し、表面温度と気圧の大きな変動を受ける可能性がある。こうした軌道は、表面上に断続的にしか水が存在し得ない劇的な季節変化をもたらすとされている。しかし、地下はそのような変化から隔離されている可能性があり、表面上もしくは表面近くの極限環境微生物は冬眠(クリプトビオシス)や超好熱菌のような適応能力を通じてこのような過酷な環境でも生き残れる可能性がある。例えば、緩歩動物(クマムシ)は脱水状態でも0.15 K(-273 ℃)[123]から424 K(151 ℃)[124]までの温度下で生き続けることができる。ハビタブルゾーンの外側を公転している惑星の表面上にいる生命は、温度が最も下がる遠点に近づくと冬眠状態になり、温度が最も十分に暖かくなる近点に近づくと活動を行うかもしれない[125]。
In the case of planets orbiting in the CHZs of red dwarf stars, the extremely close distances to the stars cause tidal locking, an important factor in habitability. For a tidally locked planet, the sidereal day is as long as the orbital period, causing one side to permanently face the host star and the other side to face away. In the past, such tidal locking was thought to cause extreme heat on the star-facing side and bitter cold on the opposite side, making many red dwarf planets uninhabitable; however, three-dimensional climate models in 2013, showed that the side of a red dwarf planet facing the host star could have extensive cloud cover, increasing its bond albedo and reducing significantly temperature differences between the two sides.[79]
Planetary-mass natural satellites have the potential to be habitable as well. However, these bodies need to fulfill additional parameters, in particular being located within the circumplanetary habitable zones of their host planets.[33] More specifically, moons need to be far enough from their host giant planets that they are not transformed by tidal heating into volcanic worlds like Io,[33] but must still remain within the Hill radius of the planet so that they are not pulled out of orbit of their host planet.[109] Red dwarfs that have masses less than 20% of that of the Sun cannot have habitable moons around giant planets, as the small size of the circumstellar habitable zone would put a habitable moon so close to the star that it would be stripped from its host planet. In such a system, a moon close enough to its host planet to maintain its orbit would have tidal heating so intense as to eliminate any prospects of habitability.[33]
A planetary object that orbits a star with high orbital eccentricity may spend only some of its year in the CHZ and experience a large variation in temperature and atmospheric pressure. This would result in dramatic seasonal phase shifts where liquid water may exist only intermittently. It is possible that subsurface habitats could be insulated from such changes and that extremophiles on or near the surface might survive through adaptions such as hibernation (cryptobiosis) and/or hyperthermostability. Tardigrades, for example, can survive in a dehydrated state temperatures between 0.150 K (−273 °C)[110] and 424 K (151 °C).[111] Life on a planetary object orbiting outside CHZ might hibernate on the cold side as the planet approaches the apastron where the planet is coolest and become active on approach to the periastron when the planet is sufficiently warm.[112]
太陽系外での発見[編集]
太陽系外惑星の中では2015年のレビューで、ケプラー62f、ケプラー186f、そしてケプラー442bの3つが潜在的に居住可能な惑星の最有力候補である可能性が高いという結論に達している[126]。これらの惑星はそれぞれ地球から1,200、490、1,120光年離れた位置にある。これらのうちケプラー186fは地球と同程度の規模で、地球の約1.2倍の大きさを持ち、赤色矮星である主星のハビタブルゾーンの外縁付近に位置している。太陽に比較的似ている恒星のハビタブルゾーン内を公転している惑星の中で、最も近傍にある地球型惑星はくじら座τ星eで、地球からは11.9光年離れている。くじら座τ星eはハビタブルゾーンの内縁付近に位置しており、推定表面温度は68 ℃となっている[127]。
ハビタブルゾーン内の地球型惑星の数を推定しようとした研究は、科学的データの有用性を反映する傾向がある。Ravi Kumar Kopparapuによる2013年の研究では、ハビタブルゾーン内に惑星が含まれる確率を示すneの値は0.48とされ[52]、これは銀河系内に約95から180億個の居住可能な惑星があるかもしれないことを意味している[128]。しかし、これはあくまで単なる統計的予測に過ぎず、こうした惑星のうち発見されているのはほんの一部である[129]。
かつて行われていた研究はより保守的なものであった。2011年に、Seth Borensteinは銀河系には生命が居住できる惑星が5億個存在すると結論付けていた[130]。NASAのジェット推進研究所による2011年の研究では、ケプラーミッションによる観測に基づいて、そのような惑星がより多く存在するとされ、スペクトル分類がF型、G型、K型の恒星の「約1.4–2.7%」がハビタブルゾーン内に惑星を持つと推定された[131][132]。
Among exoplanets, a review in 2015 came to the conclusion that Kepler-62f, Kepler-186f and Kepler-442b were likely the best candidates for being potentially habitable.[113] These are at a distance of 1200, 490 and 1,120 light-years away, respectively. Of these, Kepler-186f is similar in size to Earth with a 1.2-Earth-radius measure, and it is located towards the outer edge of the habitable zone around its red dwarf star. Among nearest terrestrial exoplanet candidates, Tau Ceti e is 11.9 light-years away. It is in the inner edge of its solar system's habitable zone, giving it an estimated average surface temperature of 68 °C (154 °F).[114]
Studies that have attempted to estimate the number of terrestrial planets within the circumstellar habitable zone tend to reflect the availability of scientific data. A 2013 study by Ravi Kumar Kopparapu put ηe, the fraction of stars with planets in the CHZ, at 0.48,[4] meaning that there may be roughly 95–180 billion habitable planets in the Milky Way.[115] However, this is merely a statistical prediction; only a small fraction of these possible planets have yet been discovered.[116]
Previous studies have been more conservative. In 2011, Seth Borenstein concluded that there are roughly 500 million habitable planets in the Milky Way.[117] NASA's Jet Propulsion Laboratory 2011 study, based on observations from the Kepler mission, raised the number somewhat, estimating that about "1.4 to 2.7 percent" of all stars of spectral class F, G, and K are expected to have planets in their CHZs.[118][119]
初期の発見[編集]
太陽系外のハビタブルゾーンで初めて惑星が発見されたのは、最初の太陽系外惑星が発見されてからわずか数年後であった。しかし、初期に発見されたこれらのような惑星はいずれも巨大なガス惑星であり、そしてその多くは楕円軌道を描いて公転していた。それにも関わらず、研究ではこれらの惑星を公転する地球ほどの規模を持つ衛星なら液体の水が存在が支えられている可能性が示されている[133]。初期の発見の一つとして、「暑すぎる」わけでもなく「寒すぎる」わけでもない温度を持つとされたため、当初「ゴルディロックス」という愛称で呼ばれていたおとめ座70番星bがある。しかし、後の研究で表面温度が金星並みに高くなっている事が示され、液体の水が存在する可能性は排除された[134]。1996年に発見されたはくちょう座16番星Bbは、軌道の一部分だけがハビタブルゾーンを通過する楕円軌道を描いており、このような軌道は極端な季節変化を起こすとされている。しかしながら、シミュレーションではその周囲に十分に大きな衛星があれば、その表面で液体の水の存在が支えられることが示唆されている[135]。
1998年に発見されたグリーゼ876bと2001年に発見されたグリーゼ876cは主星グリーゼ876のハビタブルゾーン内を公転する巨大ガス惑星で、両者ともに大きな衛星を持つかもしれない[136]。また1999年にはアンドロメダ座υ星のハビタブルゾーン内を公転するアンドロメダ座υ星dと呼ばれる別の巨大ガス惑星も発見されている。
2001年4月4日に、その存在が発表されたHD 28185 bは、軌道全体が主星のハビタブルゾーン内に位置している巨大ガス惑星で[137]、火星に匹敵するほどの低い軌道離心率を持っている[138]。HD 28185 bの周囲でそもそも最初から衛星が形成されるかは明らかではないが[139]、潮汐の相互作用により、地球質量ほどの規模を持つ居住可能な衛星を数十億年に渡って軌道上に留めれることが示唆されている[140]。
地球の約17倍の質量を持つ巨大ガス惑星であるHD 69830 dは2006年に発見され、地球から41光年離れた恒星HD 69830のハビタブルゾーン内を公転している[141]。その翌年には、かに座55番星fと呼ばれる惑星がかに座55番星Aのハビタブルゾーン内で発見されている[142][143]。十分な質量を持つ衛星がこれらの惑星の周囲に存在していれば、その表面で液体の水が存在できると考えられている[144]。
理論的にはこれらのような巨大惑星が衛星を持つことはできるが、現在の観測技術ではそのような衛星を検出することは難しく、存在が疑問視されているケプラー1625bの衛星の事例などを除いて明確に太陽系外衛星が確認されたことは未だ無い。そのため、ハビタブルゾーン内にある固体の表面を持った地球型惑星の発見は大きな関心を集めることになった。
The first discoveries of extrasolar planets in the CHZ occurred just a few years after the first extrasolar planets were discovered. However these early detections were all gas giant sized, and many in eccentric orbits. Despite this, studies indicate the possibility of large, Earth-like moons around these planets supporting liquid water.[120] One of the first discoveries was 70 Virginis b, a gas giant initially nicknamed "Goldilocks" due to it being neither "too hot" nor "too cold." Later study revealed temperatures analogous to Venus, ruling out any potential for liquid water.[121] 16 Cygni Bb, also discovered in 1996, has an extremely eccentric orbit that spends only part of its time in the CHZ, such an orbit would causes extreme seasonal effects. In spite of this, simulations have suggested that a sufficiently large companion could support surface water year-round.[122]
Gliese 876 b, discovered in 1998, and Gliese 876 c, discovered in 2001, are both gas giants discovered in the habitable zone around Gliese 876 that may also have large moons.[123] Another gas giant, Upsilon Andromedae d was discovered in 1999 orbiting Upsilon Andromidae's habitable zone.
Announced on April 4, 2001, HD 28185 b is a gas giant found to orbit entirely within its star's circumstellar habitable zone[124] and has a low orbital eccentricity, comparable to that of Mars in the Solar System.[125] Tidal interactions suggest it could harbor habitable Earth-mass satellites in orbit around it for many billions of years,[126] though it is unclear whether such satellites could form in the first place.[127]
HD 69830 d, a gas giant with 17 times the mass of Earth, was found in 2006 orbiting within the circumstellar habitable zone of HD 69830, 41 light years away from Earth.[128] The following year, 55 Cancri f was discovered within the CHZ of its host star 55 Cancri A.[129][130] Hypothetical satellites with sufficient mass and composition are thought to be able to support liquid water at their surfaces.[131]
Though in theory, such giant planets could possess moons, the technology did not exist to detect moons around them, and no extrasolar moons had been detected. Planets within the zone with the potential for solid surfaces were therefore of much greater interest.
居住可能なスーパーアース[編集]

2007年に発見されたグリーゼ581cは、ハビタブルゾーン内を公転する初めて発見されたスーパーアースであった。この発見は科学界で大きな関心を集めたが、後にグリーゼ581cは金星に似た極端な環境になっていることが後に判明した[145]。同じグリーゼ581系内で、より居住性が高いと考えられている別の惑星としてグリーゼ581dが同年に発見されているが、2014年にその存在を疑問視する研究結果も報告されている[146]。2010年にハビタブルゾーン内に発見された、また別の惑星グリーゼ581gはcとdよりも居住性が高いと考えられたが、こちらも存在は疑問視されている[147]。
The 2007 discovery of Gliese 581 c, the first super-Earth in the circumstellar habitable zone, created significant interest in the system by the scientific community, although the planet was later found to have extreme surface conditions that may resemble Venus.[132] Gliese 581 d, another planet in the same system and thought to be a better candidate for habitability, was also announced in 2007. Its existence was later disconfirmed in 2014. Gliese 581 g, yet another planet thought to have been discovered in the circumstellar habitable zone of the system, was considered to be more habitable than both Gliese 581 c and d. However, its existence was also disconfirmed in 2014.[133]

2011年8月に発見されたHD 85512 bは当初、ハビタブルゾーン内にあると推測されたが[148]、2013年にKopparapuらによって提案されたハビタブルゾーンの新たな基準に基づくと、HD 85512 bはハビタブルゾーンよりも内側を公転していることになる[129]。
2011年12月にケプラー宇宙望遠鏡によって発見された惑星ケプラー22bは、初めて太陽に似た恒星の周囲で発見された、主星の手前を通過する太陽系外惑星であった[149]。大きさは地球の約2倍で、海洋惑星である可能性が示されている[150]。2011年に発見され、その翌年にその存在が発表されたグリーゼ667Ccは、主星グリーゼ667Cのハビタブルゾーン内を公転するスーパーアースである[151]。
2012年9月に、地球から約49光年離れた赤色矮星のグリーゼ163[152]のハビタブルゾーン内を公転しているグリーゼ163cが発見された。グリーゼ163cは少なくとも地球の6.9倍の質量を持ち、大きさは地球の1.8–2.4倍と推定されている。主星から地球よりも約40%多い放射線を受けているので、表面温度は約60 ℃とされている[153][154][155]。2012年11月に暫定的に発見された惑星候補HD 40307 gは、主星HD 40307のハビタブルゾーン内を公転している[156]。2012年12月には、約12光年離れている太陽に似た恒星くじら座τ星のハビタブルゾーン内を公転するくじら座τ星eとくじら座τ星fと呼ばれる2つの惑星が発見された[157]。質量は地球よりも大きいが、現在までに知られているハビタブルゾーン内を公転する惑星の中では最も質量が小さい惑星の一つである[158]。しかし、外側を公転しているくじら座τ星fはHD 85512 bと同様に2013年にKopparapuらによって提案されたハビタブルゾーンの基準に基づくと、ハビタブルゾーン内には存在していないことになる[159]。
Discovered in August 2011, HD 85512 b was initially speculated to be habitable,[134] but the new circumstellar habitable zone criteria devised by Kopparapu et al. in 2013 place the planet outside the circumstellar habitable zone.[116]
Kepler-22 b, discovered in December 2011 by the Kepler space probe,[135] is the first transiting exoplanet discovered around a Sun-like star. With a radius 2.4 times that of Earth, Kepler-22b has been predicted by some to be an ocean planet.[136] Gliese 667 Cc, discovered in 2011 but announced in 2012,[137] is a super-Earth orbiting in the circumstellar habitable zone of Gliese 667 C.
Gliese 163 c, discovered in September 2012 in orbit around the red dwarf Gliese 163[138] is located 49 light years from Earth. The planet has 6.9 Earth masses and 1.8–2.4 Earth radii, and with its close orbit receives 40 percent more stellar radiation than Earth, leading to surface temperatures of about 60° C.[139][140][141] HD 40307 g, a candidate planet tentatively discovered in November 2012, is in the circumstellar habitable zone of HD 40307.[142] In December 2012, Tau Ceti e and Tau Ceti f were found in the circumstellar habitable zone of Tau Ceti, a Sun-like star 12 light years away.[143] Although more massive than Earth, they are among the least massive planets found to date orbiting in the habitable zone;[144] however, Tau Ceti f, like HD 85512 b, did not fit the new circumstellar habitable zone criteria established by the 2013 Kopparapu study.[145]
地球に近い大きさの惑星とソーラーアナログ[編集]


最近では、大きさや質量が地球に似ていると考えられている惑星も発見されるようになってきた。「地球規模(Earth-sized)」といえる惑星の範囲は、通常は質量によって定義されている。一般的に1.9地球質量が「スーパーアースクラス」の定義域の下限として用いられることが多く、一方で、地球よりも小さい惑星の分類である「サブアースクラス(sub-Earth class)」は金星質量(0.815地球質量)以下の範囲となる。半径が1.5地球半径を超える場合、中心部のにある岩石質の核より上にある体積の大部分が揮発性の物質になるため[160]、半径が大きくなると惑星の平均密度が急速に小さくなる。これを考慮して地球規模の惑星の上限半径を1.5地球半径とすることもある。とても地球に似ている惑星の分類であるアースアナログ(もしくはアースツイン)に分類されるには、大きさや質量以上に多くの条件を満たす必要がある。しかし、そのような特性を観測することは現在の技術では不可能である。
太陽に似ている恒星はソーラーアナログ(またはソーラーツイン)に分類される。今日までに、太陽と特性が完全に一致する太陽の「双子星」はまだ知られていないが、特性がほとんど一致する太陽の「双子星」と考えられる恒星がいくつか存在している。太陽と同じG2V型のスペクトル分類、5,778 Kの表面温度、一致した金属量を持ち、形成から約46億年が経過しており、光度の変化が0.1%になっている恒星が正確な太陽の「双子星」となる[161]。形成から46億年が経過した恒星は最も安定した状態にある。適切な金属量や大きさもまた、小さな光度変化ことに対して非常に重要なものとなる[162][163][164]。
Recent discoveries have uncovered planets that are thought to be similar in size or mass to Earth. "Earth-sized" ranges are typically defined by mass. The lower range used in many definitions of the super-Earth class is 1.9 Earth masses; likewise, sub-Earths range up to the size of Venus (~0.815 Earth masses). An upper limit of 1.5 Earth radii is also considered, given that above 1.5 R⊕ the average planet density rapidly decreases with increasing radius, indicating these planets have a large fraction of volatiles by volume overlying a rocky core.[146] A truly Earth-like planet, an Earth analog or "Earth twin", would need to meet many conditions beyond size and mass; such properties are not observable using current technology.
A solar analog (or "solar twin") is a star that resembles the Sun. To date no solar twin with an exact match as that of the Sun has been found, however, there are some stars that are nearly identical to the Sun, and are such considered solar twins. An exact solar twin would be a G2V star with a 5,778 K temperature, be 4.6 billion years old, with the correct metallicity and a 0.1% solar luminosity variation.[147] Stars with an age of 4.6 billion years are at the most stable state. Proper metallicity and size are also very important to low luminosity variation.[148][149][150]
NASAのケプラー宇宙望遠鏡とW・M・ケック天文台によって収集されたデータから、銀河系内に含まれる太陽のような恒星の22%がそのハビタブルゾーン内に地球規模の惑星を持つと推定されている[165]。
2013年1月7日、ケプラーのミッションチームに属する天文学者たちは、太陽に似た恒星ケプラー69を公転する、地球の1.7倍の大きさを持つ地球規模の太陽系外惑星候補ケプラー69c(KOI-172.02)の発見を発表した。この惑星はハビタブルゾーン内にあり、居住に適した環境になっていると予想された[166][167][168][169]。しかし、現在では暴走温室効果により表面は金星のような環境になっていると考えられている[170]。同年4月19日には、ケプラーチームはケプラー62のハビタブルゾーン内を公転する2つの惑星の発見が発表された。これらの惑星はケプラー62eとケプラー62fと呼ばれており、それぞれ地球の1.6倍と1.4倍の大きさを持つ[166][167][171]。
2014年4月に発見が発表された地球の1.1倍の大きさを持つ惑星ケプラー186fは、質量が知られておらず、主星もソーラーアナログではないにも関わらず、トランジット法によって発見された最も地球に大きさが近い惑星である[172][173][174]。
2014年6月に発見されたカプタインbは12.8光年離れた位置にある赤色矮星カプタイン星のハビタブルゾーン内を公転しており、地球の約4.8倍の質量を持つ岩石惑星で、半径は地球の1.5倍ほどと推定されている[175]。しかし、2015年には存在を疑問視する研究結果も報告されている[176]。
Using data collected by NASA's Kepler Space observatory and the W. M. Keck Observatory, scientists have estimated that 22% of solar-type stars in the Milky Way galaxy have Earth-sized planets in their habitable zone.[151]
On 7 January 2013, astronomers from the Kepler team announced the discovery of Kepler-69c (formerly KOI-172.02), an Earth-size exoplanet candidate (1.7 times the radius of Earth) orbiting Kepler-69, a star similar to our Sun, in the CHZ and expected to offer habitable conditions.[152][153][154][155] The discovery of two planets orbiting in the habitable zone of Kepler-62, by the Kepler team was announced on April 19, 2013. The planets, named Kepler-62e and Kepler-62f, are likely solid planets with sizes 1.6 and 1.4 times the radius of Earth, respectively.[154][155][156]
With a radius estimated at 1.1 Earth, Kepler-186f, discovery announced in April 2014, is the closest yet size to Earth of an exoplanet confirmed by the transit method[157][158][159] though its mass remains unknown and its parent star is not a Solar analog.
Kapteyn b, discovered in June 2014 is a possible rocky world of about 4.8 Earth masses and about 1.5 earth radii was found orbiting the habitable zone of the red subdwarf Kapteyn's Star, 12.8 light-years away.[160]
2015年1月6日、NASAはケプラー宇宙望遠鏡によって発見された太陽系外惑星が1,000に達したと発表した。それと同時にハビタブルゾーン内を公転する新たな3つの惑星ケプラー438b・ケプラー440b・ケプラー442bが発表された[177]。そのうちケプラー438bとケプラー442bは地球に近い大きさで、おそらく岩石から構成されている[177]。残るケプラー440bはスーパーアースとされている。同年1月16日に発見が発表された惑星K2-3dは地球の約1.5倍の半径を持ち、主星K2-3のハビタブルゾーン内を公転しており、地球よりも1.4倍多くの可視光放射を受けていることが判明している[178]。
2015年7月23日に発見が発表されたケプラー452bは地球よりも50%大きく、おそらく岩石から成るとされている。G型星(ソーラーアナログ)に分類される恒星ケプラー452のハビタブルゾーン内を385日かけて公転している[179][180]。主星や軌道要素が地球のものと似ているため、発表において「Earth 2.0」や「地球のいとこ」といった表現が用いられた[181]。
2016年7月、227光年離れた位置にある赤色矮星K2-72の周囲を公転している潜在的に居住可能な2つの惑星が、ケプラーの延長ミッションであるK2ミッションでの観測で発見された。これらの惑星はK2-72dとK2-72eと呼ばれ、両社共に地球とほぼ同じ大きさで、主星から受ける放射量もほぼ同等である[182]。

2017年2月には、約40光年離れた超低温矮星TRAPPIST-1のハビタブルゾーン内を、すでに2016年5月に存在が発表されていた惑星を含めて複数の惑星が公転していることが判明したと発表された[183]。
2017年4月20日に発見が発表された高密度惑星LHS 1140bは、地球の6.6倍の質量と1.4倍の半径を持ち、主星のLHS 1140は太陽の15%ほどの質量で、大部分の赤色矮星よりもフレアなどの恒星活動が弱い[184]。LHS 1140bはトランジット法とドップラー分光法(視線速度法)の両方によって検出されている数少ない惑星で、大気を観測できる可能性がある。
2017年6月にドップラー分光法で発見された、地球の約3倍の質量を持つ惑星ルイテンbは、12.2光年離れた位置にあるルイテン星のハビタブルゾーン内を公転している[185]。
11光年離れた位置にある、恒星活動が比較的静かな赤色矮星ロス128の10年間に渡る視線速度のデータの調査により、2017年11月に惑星ロス128bが発見された。地球の1.35倍の質量を持ち、地球規模の大きさで岩石で構成されていると考えられている[186]。
2018年3月に発見された惑星K2-155dは、地球の1.64倍の半径を持った岩石惑星と考えられており、203光年離れた赤色矮星のハビタブルゾーン内を公転している[187][188][189]。
2019年6月、12.5光年離れた位置にある暗い赤色矮星ティーガーデン星のハビタブルゾーン内に地球とほぼ同等の質量を持つ2つの惑星が発見されたと発表された[190]。
On 6 January 2015, NASA announced the 1000th confirmed exoplanet discovered by the Kepler Space Telescope. Three of the newly confirmed exoplanets were found to orbit within habitable zones of their related stars: two of the three, Kepler-438b and Kepler-442b, are near-Earth-size and likely rocky; the third, Kepler-440b, is a super-Earth.[161] Announced 16 January, K2-3d a planet of 1.5 Earth radii was found orbiting within the habitable zone of K2-3, receiving 1.4 times the intensity of visible light as Earth.[162]
Kepler-452b, announced on 23 July 2015 is 50% bigger than Earth, likely rocky and takes approximately 385 Earth days to orbit the habitable zone of its G-class (solar analog) star Kepler-452.[163][164]
The discovery of a system of three tidally-locked planets orbiting the habitable zone of an ultracool dwarf star, TRAPPIST-1, was announced in May 2016.[165] The discovery is considered significant because it greatly increases the possibility of smaller, cooler, more numerous and closer stars possessing habitable planets.
Two potentially habitable planets, discovered by the K2 mission in July 2016 orbiting around the M dwarf K2-72 around 227 light year from the Sun: K2-72c and K2-72e are both of similar size to Earth and receive similar amounts of stellar radiation.[166]
Announced on the 20 April 2017, LHS 1140b is a super-dense super-Earth 39 light years away, 6.6 times Earth's mass and 1.4 times radius, its star 15% the mass of the Sun but with much less observable stellar flare activity than most M dwarfs.[167] The planet is one of few observable by both transit and radial velocity that's mass is confirmed with an atmosphere may be studied.
Discovered by radial velocity in June 2017, with approximately 3 times the mass of Earth, Luyten b orbits within the habitable zone of Luyten's Star just 12.2 light years away.[168]
At 11 light-years away, a second closest planet, Ross 128 b, was announced in November 2017 following a decade's radial velocity study of relatively "quiet" red dwarf star Ross 128. At 1.35 Earth's mass is it roughly Earth sized and likely rocky in composition.[169]
Discovered in March 2018, K2-155d is about 1.64 time the radius of Earth, is likely rocky and orbits in the habitable zone of its red dwarf star 203 light years away.[170][171][172]
One of the earliest discoveries by the Transiting Exoplanet Survey Satellite (TESS) announced July 31, 2019 is a Super Earth planet GJ 357 d orbiting the outer edge of a red dwarf 31 light years away.[173]
ケプラーが発見した注目の太陽系外惑星 |
---|
![]() (ケプラー62e・ケプラー62f・ケプラー155c・ケプラー186f・ケプラー235e・ケプラー283c・ケプラー296e・ケプラー296f・ケプラー438b・ケプラー440b・ケプラー442b・ケプラー452b)[177] |
ハビタブルゾーン外での居住性[編集]

液体の水がある環境は、大気圧が無い場合やハビタブルゾーンの領域外でも存在できることがわかっている。例えば、土星の衛星であるタイタンやエンケラドゥス、そして木星の衛星であるエウロパやガニメデは、地下に大量の水をたたえた海を持っているかもしれない[191]。
ハビタブルゾーンの外では、潮汐加熱と放射性崩壊の2つが液体の水の存在に寄与できる可能性のある熱源である[22][23]。2011年にAbbotとSwitzerhaは、放射性崩壊による加熱と厚い表層の氷による断熱の結果として、地下水が自由浮遊惑星に存在する可能性を提唱した[25]。
地球上の生命体は、実際には安定した地下の生息地から発生したのではないかという理論もあり[192][193]、これらのような地下の湿った地球外生命体の生息地が「生命で溢れる」ようになるのは一般的なものかもしれない[194]。実際に地球上でも、表面から6 km以上深いところで微生物を発見できる可能性がある[195]。
もう一つ考えられる可能性として、ハビタブルゾーン外の天体に生息する生命体が水を全く必要としない代わりの生化学を用いるかもしれないということが挙げられる。宇宙生物学者のChristopher McKayは、メタン(CH4)が「氷の生物(Cryolife)」の進化を促す溶媒になる可能性があることを示しており、太陽系における「メタンのハビタブルゾーン」は太陽から11 au(約16億1000万 km)離れた領域にある[28]。土星の衛星タイタンはこのメタンのハビタブルゾーン付近に位置しており、炭化水素の湖の存在や大気からメタンの雨が降ることから、タイタンはMcKayが提案した「氷の生物」を発見するのに理想的な環境となっている[28]。さらに、微生物実験ではいくつかの条件下ならハビタブルゾーン外でも生命体が生存可能であることが判明している[196]。
Liquid-water environments have been found to exist in the absence of atmospheric pressure, and at temperatures outside the CHZ temperature range. For example, Saturn's moons Titan and Enceladus and Jupiter's moons Europa and Ganymede, all of which are outside the habitable zone, may hold large volumes of liquid water in subsurface oceans.[174]
Outside the CHZ, tidal heating and radioactive decay are two possible heat sources that could contribute to the existence of liquid water.[15][16] Abbot and Switzer (2011) put forward the possibility that subsurface water could exist on rogue planets as a result of radioactive decay-based heating and insulation by a thick surface layer of ice.[18]
With some theorising that life on Earth may have actually originated in stable, subsurface habitats,[175][176] it has been suggested that it may be common for wet subsurface extraterrestrial habitats such as these to 'teem with life'.[177] Indeed, on Earth itself living organisms may be found more than 6 kilometres below the surface.[178]
Another possibility is that outside the CHZ organisms may use alternative biochemistries that do not require water at all. Astrobiologist Christopher McKay, has suggested that methane (CH 4) may be a solvent conducive to the development of "cryolife", with the Sun's "methane habitable zone" being centered on 1,610,000,000 km (1.0×109 mi; 11 AU) from the star.[21] This distance is coincident with the location of Titan, whose lakes and rain of methane make it an ideal location to find McKay's proposed cryolife.[21] In addition, testing of a number of organisms has found some are capable of surviving in extra-CHZ conditions.[179]
複雑な知的生命体にとっての意義[編集]
レアアース仮説では複雑で知的な生命体は非常に稀であり、ハビタブルゾーンはそれにおいて重要な要素の一つであると主張している。2004年にWardとBrownleeが出版した書籍によると、ハビタブルゾーンと天体の表面にある液体の水は生命体を維持するための主要な要素だけでなく、多細胞生物の出現と進化に必要な二次的条件を支えるための要素でもあるとされている。居住性をもたらすには、地質学(プレートテクトニクスの維持における液体の水の役割)[197]と生化学(大気の酸素化に必要な光合成を支える放射エネルギーの役割)[198]の両方の二次的要素が必要となる。しかし一方で、2002年にイアン・スチュアートとJack Cohenが記した著書「Evolving the Alien」では知的生命体はハビタブルゾーンの外側でも出現し得ると主張している[199]。ハビタブルゾーン外での知的生命体は代わりの生化学どころか[199]、核反応からでさえ[200]、地下環境で進化する可能性がある。
地球上では、いくつかの複雑な多細胞生物(または真核生物)がハビタブルゾーン外の状態を乗り切れる可能性があることが確認されている。地熱エネルギーは古代の迂回的な生態系を維持し、Riftia pachyptila(シボグリヌム科)のような複雑で大型の生命体の存在を支えている[201]。ハビタブルゾーンの外側にある、エウロパやエンケラドゥスのような固体の地殻の下で加熱された海でも同様の環境になっているかもしれない[202]。真核生物を含む多数の微生物が擬似条件下、および地球低軌道上で研究実験が行われている。例としてオニクマムシは、水の沸点を超えるような極端に高い温度下や冷たい真空の宇宙空間でも耐えることができる[203]。さらに、チズゴケやXanthoria elegans(チャシブゴケ菌綱)といった植物は、表面に液体の水が存在するには不十分なほど薄い大気圧下や、放射エネルギーがほとんどの植物が光合成に必要とする量よりもはるかに少ない環境下でも生存することが判明している[204][205][206]。菌類のCryomyces antarcticusやDothideomycetes(共にクロイボタケ綱)もまた、火星のような環境下でも生存して繁殖することができる[206]。
The Rare Earth hypothesis argues that complex and intelligent life is uncommon and that the CHZ is one of many critical factors. According to Ward & Brownlee (2004) and others, not only is a CHZ orbit and surface water a primary requirement to sustain life but a requirement to support the secondary conditions required for multicellular life to emerge and evolve. The secondary habitability factors are both geological (the role of surface water in sustaining necessary plate tectonics)[31] and biochemical (the role of radiant energy in supporting photosynthesis for necessary atmospheric oxygenation).[180] But others, such as Ian Stewart and Jack Cohen in their 2002 book Evolving the Alien argue that complex intelligent life may arise outside the CHZ.[181] Intelligent life outside the CHZ may have evolved in subsurface environments, from alternative biochemistries[181] or even from nuclear reactions.[182]
On Earth, several complex multicellular life forms (or eukaryotes) have been identified with the potential to survive conditions that might exist outside the conservative habitable zone. Geothermal energy sustains ancient circumvental ecosystems, supporting large complex life forms such as Riftia pachyptila.[183] Similar environments may be found in oceans pressurised beneath solid crusts, such as those of Europa and Enceladus, outside of the habitable zone.[184] Numerous microorganisms have been tested in simulated conditions and in low Earth orbit, including eukaryotes. An animal example is the Milnesium tardigradum, which can withstand extreme temperatures well above the boiling point of water and the cold vacuum of outer space.[185] In addition, the plants Rhizocarpon geographicum and Xanthoria elegans have been found to survive in an environment where the atmospheric pressure is far too low for surface liquid water and where the radiant energy is also much lower than that which most plants require to photosynthesize.[186][187][188] The fungi Cryomyces antarcticus and Cryomyces minteri are also able to survive and reproduce in Mars-like conditions.[188]
ヒトを含む動物認識を持つことが知られている種は大量のエネルギーを必要とし[207]、大気中に豊富に含まれている酸素と放射エネルギーから合成された多くの化学エネルギーの利用可能性を含む特定の条件下に適応している。人類が他の惑星を植民化するのであれば、ハビタブルゾーン内にある真のアースアナログは地球に最も自然環境が近い生息地をもたらす惑星となる可能性が高い。この概念は1964年のStephen H. Doleによる研究で基礎づけられている。惑星に適切な温度、重力、大気圧、そして水があれば、宇宙服やスペースコロニーの必要性が排除され、地球上の複雑な生命体がその惑星で繁栄する可能性がある[37]。
ハビタブルゾーン内にある惑星は、地球以外の場所で知的生命体を探している研究者にとっては依然として最も重要な関心事となっている[208]。この銀河系内にある知的文明の数を推定するために時々使用されるドレイクの方程式では、各恒星のハビタブルゾーンを公転する惑星質量天体の平均数を示すneという因子が含まれている。この値が低いとレアアース仮説を支持するものになり、知的生命体が宇宙では珍しいものであると仮定される。逆に値が高いとニコラウス・コペルニクスが唱えたコペルニクスの原理(平凡の原理)の根拠を示すものとなる[41]。1971年のフランク・ドレイクとバーナード・オリバーによるNASAの報告では、ヒドロキシ基と水の成分である水素のスペクトルにおける吸収線に基づいた「水の穴(Water hole)」を地球外生命体とのコミュニケーション手段に明白かつ適切なバンドとして提案し[209][210]、それ以来この提案は地球外知的生命体の探求に関わる天文学者たちによって広く採用されてきた。Jill TarterやMargaret Turnbullなどによれば、ハビタブルゾーン候補は「狭い滝壺」を探すための最優先目標であるとしている[211][212]。
ハビタブルゾーンは複雑な生命体の生息地として最も可能性が高い領域と考えらているので、アクティブSETI(METI)での取り組みは惑星を持つ可能性が高い惑星系に焦点を当てている。例えば2001年に送信された電波信号ティーンエイジメッセージ(Teen Age Message)と2003年に送信されたコズミックコール2(Cosmic Call 2)は、木星規模の惑星を3つ持ち、ハビタブルゾーン内に地球型惑星を持つ可能性があるおおぐま座47番星系に向かって発信された[213][214][215][216]。ティーンエイジメッセージはハビタブルゾーン内に巨大ガス惑星を持つかに座55番星にも発信された[142]。2008年に送信されたメッセージフロムアース(A Message From Earth)[217]および2009年に送信されたハローフロムアース(Hello From Earth)は、ハビタブルゾーン内にcとd、そして未確認のgの3つの惑星を持つグリーゼ581系に発信された。
Species, including humans, known to possess animal cognition require large amounts of energy,[189] and have adapted to specific conditions, including an abundance of atmospheric oxygen and the availability of large quantities of chemical energy synthesized from radiant energy. If humans are to colonize other planets, true Earth analogs in the CHZ are most likely to provide the closest natural habitat; this concept was the basis of Stephen H. Dole's 1964 study. With suitable temperature, gravity, atmospheric pressure and the presence of water, the necessity of spacesuits or space habitat analogues on the surface may be eliminated and complex Earth life can thrive.[2]
Planets in the CHZ remain of paramount interest to researchers looking for intelligent life elsewhere in the universe.[190] The Drake equation, sometimes used to estimate the number of intelligent civilizations in our galaxy, contains the factor or parameter ne, which is the average number of planetary-mass objects orbiting within the CHZ of each star. A low value lends support to the Rare Earth hypothesis, which posits that intelligent life is a rarity in the Universe, whereas a high value provides evidence for the Copernican mediocrity principle, the view that habitability—and therefore life—is common throughout the Universe.[31] A 1971 NASA report by Drake and Bernard Oliver proposed the "water hole", based on the spectral absorption lines of the hydrogen and hydroxyl components of water, as a good, obvious band for communication with extraterrestrial intelligence[191][192] that has since been widely adopted by astronomers involved in the search for extraterrestrial intelligence. According to Jill Tarter, Margaret Turnbull and many others, CHZ candidates are the priority targets to narrow waterhole searches[193][194] and the Allen Telescope Array now extends Project Phoenix to such candidates.[195]
Because the CHZ is considered the most likely habitat for intelligent life, METI efforts have also been focused on systems likely to have planets there. The 2001 Teen Age Message and the 2003 Cosmic Call 2, for example, were sent to the 47 Ursae Majoris system, known to contain three Jupiter-mass planets and possibly with a terrestrial planet in the CHZ.[196][197][198][199] The Teen Age Message was also directed to the 55 Cancri system, which has a gas giant in its CHZ.[129] A Message from Earth in 2008,[200] and Hello From Earth in 2009, were directed to the Gliese 581 system, containing three planets in the CHZ—Gliese 581 c, d, and the unconfirmed g.
その他のハビタブルゾーン[編集]
銀河系のハビタブルゾーン (GHZ)[編集]

銀河の中心から十分に近いため重い元素が高レベルに存在し、そのため地球型惑星が形成されるのに十分だが、しかし中心から十分遠くでもあり中心付近での高い恒星密度による彗星や小惑星の衝突の危険、超新星爆発による放射線、さらに銀河中心のブラックホールの影響などから逃れられる銀河内における領域を銀河系のハビタブルゾーン(英語: Galactic habitable zone、GHZ)と呼ぶことがある[41][11]。すなわち、銀河のなかでどこに惑星系のハビタブルゾーンがそもそも存在できる条件なのか、この点を考慮したものである。
銀河系におけるハビタブルゾーンは、内縁が銀河核周辺で外縁が中心から10,000パーセク(約32,600光年)離れた環状の範囲で、形成されてから40–80億年が経過した星々を含み、時間が経過するごとにゆっくりと広がっていくとされている[11][218][219]。他の銀河のハビタブルゾーンについてはほとんど分かっていないが、約250万光年離れているアンドロメダ銀河のハビタブルゾーンは銀河中心から3,000–7,000パーセク(9,780–22,820光年)離れた領域にある、形成から60–70億年が経過した恒星から成るという研究もある[220]。
銀河系のハビタブルゾーンについてはまだ研究が進んでおらず、生物に対する超新星などによる放射線の影響はまだ解明されていない。研究では重い元素や金属性物質が非常に多く、恒星が近い軌道に巨大な惑星を保持していると見られる領域が存在することも明らかにされている。このような巨大惑星をもつ惑星系では、生命が誕生する可能性を持つ地球クラスの惑星を破壊するかもしれない。これらの理由から、銀河系のハビタブルゾーンを決定するには不確かなことが多いため、明確な定義付けはできない可能性がある。
ブラックホールのハビタブルゾーン[編集]

銀河系のハビタブルゾーンの概念では、中心に存在する超大質量ブラックホール(いて座A*)により銀河系の中心付近は生命体には適しないとされてきた。しかし、2019年5月にこの超大質量ブラックホールの周りで比較的生命体の存在が維持できる領域、すなわちブラックホールのハビタブルゾーンが存在するという研究結果が、ハーバード大学の研究グループによって発表された[12][13]。
超大質量ブラックホールの周囲には吸い込んだ物質から成る降着円盤が形成される。この降着円盤は周囲にX線などの強い電磁波を発しており、これまでの研究ではいて座A*クラスの質量を持つ超大質量ブラックホールの場合、ブラックホールから3,200光年以内の範囲にある惑星は放出された電磁波により大気が消失してしまうと考えられていた[12][13]。しかし、ハーバード大学のグループによる研究によると、実際にこうした超大質量ブラックホールの周りで電磁波の影響が及ぶのは100光年以内になることが判明し、さらにブラックホールの降着円盤から放出された電磁波の量が、惑星の大気を失わせるほど多くなく、逆に生命体が誕生するのに必要な有機物や化合物の反応が進まなくなるほど少なくもない、丁度良いバランスが取れる領域があることが判明し、研究グループはこれがブラックホールのハビタブルゾーンであるとしている[12][13]。いて座A*の場合、140光年離れた領域がいて座A*のハビタブルゾーンになると推定されている[12][13]。
脚注[編集]
注釈[編集]
出典[編集]
- ^ a b c d “ハビタブルゾーン”. 天文学辞典. 日本天文学会. 2019年7月23日閲覧。
- ^ a b c “ハビタブルゾーンとは - コトバンク”. コトバンク. 2019年7月23日閲覧。
- ^ “ハビタブルゾーンに地球型の3惑星発見”. ナショナルジオグラフィック (2013年4月22日). 2016年6月12日閲覧。
- ^ a b c d 小玉貴則 (2018年). “系外惑星「遠い世界の物語」 その10 ~ハビタブル惑星の現状とこれから” (PDF). 日本惑星科学会. 2019年7月23日閲覧。
- ^ “立方体地球 -Cubic Earth- ハビタブルゾーンってどこなの?”. 公益財団法人日本科学協会. 2019年12月8日閲覧。
- ^ a b 長沼・井田 2014, pp. 92–96.
- ^ Cruz, Maria; Coontz, Robert (2013). “Exoplanets - Introduction to Special Issue”. Science 340 (6132): 565. doi:10.1126/science.340.6132.565. PMID 23641107 .
- ^ National Academies of Sciences, Engineering, and Medicine (2018-10-10). “An Astrobiology Strategy for the Search for Life in the Universe”. National Academies of Sciences, Engineering, and Medicine (The National Academies Press). doi:10.17226/25252. ISBN 978-0-309-48419-0 .
- ^ a b c Lammer, H.; Bredehöft, J. H.; Coustenis, A.; Khodachenko, M. L. et al. (2009). “What makes a planet habitable?” (PDF). The Astronomy and Astrophysics Review 17 (2): 181–249. Bibcode: 2009A&ARv..17..181L. doi:10.1007/s00159-009-0019-z. オリジナルの2016-06-02時点におけるアーカイブ。 .
- ^ Schirber, Michael (2009年10月26日). “Detecting Life-Friendly Moons”. Astrobiology Magazine. 2019年7月23日閲覧。
- ^ a b c Gowanlock, M. G.; Patton, D. R.; McConnell, S. M. (2011). “A Model of Habitability Within the Milky Way Galaxy”. Astrobiology 11 (9): 855–873. arXiv:1107.1286. Bibcode: 2011AsBio..11..855G. doi:10.1089/ast.2010.0555. PMID 22059554.
- ^ a b c d e 松村武宏 (2019年6月24日). “超大質量ブラックホール周囲でも生命が存在できる可能性”. sorae.info. 2019年7月23日閲覧。
- ^ a b c d e Mara Johnson-Groh (2019年6月19日). “Voracious Black Holes Could Feed Alien Life on Rogue Worlds”. 2019年7月23日閲覧。
- ^ a b c d e f Hadhazy, Adam (2013年4月3日). “The 'Habitable Edge' of Exomoons”. Astrobiology Magazine (NASA) 2019年7月23日閲覧。
- ^ “地上に生まれた最初の生命 他の星に生命体の可能性”. NIKKEI STYLE (2015年12月20日). 2018年1月19日閲覧。
- ^ Overbye, Dennis (2015年1月6日). “As Ranks of Goldilocks Planets Grow, Astronomers Consider What's Next”. The New York Times 2019年7月23日閲覧。
- ^ Overbye, Dennis (2013年11月4日). “Far-Off Planets Like the Earth Dot the Galaxy”. The New York Times 2019年7月23日閲覧。
- ^ a b Petigura, Eric A.; Howard, Andrew W.; Marcy, Geoffrey W. (2013). “Prevalence of Earth-size planets orbiting Sun-like stars”. Proceedings of the National Academy of Sciences of the United States of America 110 (48): 19273–19278. arXiv:1311.6806. Bibcode: 2013PNAS..11019273P. doi:10.1073/pnas.1319909110. PMC 3845182. PMID 24191033 .
- ^ Khan, Amina (2013年11月4日). “Milky Way may host billions of Earth-size planets”. Los Angeles Times 2019年7月23日閲覧。
- ^ Anglada-Escudé, Guillem et al. (2016). “A terrestrial planet candidate in a temperate orbit around Proxima Centauri”. Nature 536 (7617): 437–440. arXiv:1609.03449. Bibcode: 2016Natur.536..437A. doi:10.1038/nature19106. PMID 27558064.
- ^ Edwards, Katrina J.; Becker, Keir; Colwell, Frederick (2012). “The Deep, Dark Energy Biosphere: Intraterrestrial Life on Earth”. Annual Review of Earth and Planetary Sciences 40 (1): 551–568. Bibcode: 2012AREPS..40..551E. doi:10.1146/annurev-earth-042711-105500. ISSN 0084-6597.
- ^ a b Cowen, Ron (2008年6月7日). “A Shifty Moon”. Science News 2019年7月23日閲覧。
- ^ a b Bryner, Jeanna (2009年6月24日). “Ocean Hidden Inside Saturn's Moon”. Space.com. TechMediaNetwork 2019年7月23日閲覧。
- ^ Abbot, D. S.; Switzer, E. R. (2011). “The Steppenwolf: A Proposal for a Habitable Planet in Interstellar Space”. The Astrophysical Journal 735 (2): L27. arXiv:1102.1108. Bibcode: 2011ApJ...735L..27A. doi:10.1088/2041-8205/735/2/L27.
- ^ a b “Rogue Planets Could Harbor Life in Interstellar Space, Say Astrobiologists”. MIT Technology Review. MIT Technology Review. (2011年2月9日) 2019年7月23日閲覧。
- ^ Wall, Mike (2015年9月28日). “Salty Water Flows on Mars Today, Boosting Odds for Life”. Space.com 2019年7月23日閲覧。
- ^ Sun, Jiming; Clark, Bryan K.; Torquato, Salvatore; Car, Roberto (2015). “The phase diagram of high-pressure superionic ice”. Nature Communications 6: 8156. Bibcode: 2015NatCo...6E8156S. doi:10.1038/ncomms9156. ISSN 2041-1723. PMC 4560814. PMID 26315260 .
- ^ a b c d Villard, Ray (2011年11月18日). “Alien Life May Live in Various Habitable Zones : Discovery News”. Discovery Communications LLC. News.discovery.com 2019年7月23日閲覧。
- ^ 3rd Edition (1728), trans Bruce, I
- ^ Huggett, Richard J. (1995). Geoecology: An Evolutionary Approach. Routledge, Chapman & Hall. p. 10. ISBN 978-0-415-08689-9
- ^ Strughold, Hubertus (1953). The Green and Red Planet: A Physiological Study of the Possibility of Life on Mars. University of New Mexico Press
- ^ “Member Directory | Harlow Shapley” [会員検索 | ハーロー・シャプレー] (英語). 米国科学アカデミー. 2019年12月13日閲覧。
- ^ Kasting, James (2010). How to Find a Habitable Planet. Princeton University Press. p. 127. ISBN 978-0-691-13805-3
- ^ a b c d e f Kasting, James F.; Whitmire, Daniel P.; Reynolds, Ray T. (1993). “Habitable Zones around Main Sequence Stars”. Icarus 101 (1): 108–118. Bibcode: 1993Icar..101..108K. doi:10.1006/icar.1993.1010. PMID 11536936 .
- ^ Huang, Su-Shu (1966). Extraterrestrial life: An Anthology and Bibliography. National Research Council (U.S.). Study Group on Biology and the Exploration of Mars. Washington, D. C.: National Academy of Sciences. pp. 87–93
- ^ Huang, Su-Shu (1960). “Life-Supporting Regions in the Vicinity of Binary Systems”. Publications of the Astronomical Society of the Pacific 72 (425): 106–114. Bibcode: 1960PASP...72..106H. doi:10.1086/127489.
- ^ a b c d Dole, Stephen H. (1964). Habitable Planets for Man. Blaisdell Publishing Company. p. 103
- ^ Gilster, Paul (2004). Centauri Dreams: Imagining and Planning Interstellar Exploration. Springer. p. 40. ISBN 978-0-387-00436-5
- ^ "The Goldilocks Zone" (Press release). NASA. 2 October 2003. 2019年7月23日閲覧。
- ^ Seager, Sara (2013). “Exoplanet Habitability”. Science 340 (577): 577–581. Bibcode: 2013Sci...340..577S. doi:10.1126/science.1232226. PMID 23641111.
- ^ a b c d Brownlee, Donald; Ward, Peter (2004). Rare Earth: Why Complex Life Is Uncommon in the Universe. New York: Copernicus. ISBN 978-0-387-95289-5
- ^ Gonzalez, Guillermo; Brownlee, Donald; Ward, Peter (2001). “The Galactic Habitable Zone I. Galactic Chemical Evolution”. Icarus 152 (1): 185–200. arXiv:astro-ph/0103165. Bibcode: 2001Icar..152..185G. doi:10.1006/icar.2001.6617.
- ^ “潮汐加熱”. 天文学辞典. 公益社団法人日本天文学会. 2019年12月13日閲覧。
- ^ 長沼・井田 2014, pp. 104–109.
- ^ a b Fogg, M. J. (1992). “An Estimate of the Prevalence of Biocompatible and Habitable Planets”. Journal of the British Interplanetary Society 45 (1): 3–12. Bibcode: 1992JBIS...45....3F. PMID 11539465.
- ^ a b Redd, Nola Taylor (2011年8月25日). “Greenhouse Effect Could Extend Habitable Zone”. Astrobiology Magazine. NASA. 2019年7月23日閲覧。
- ^ a b 長沼・井田 2014, pp. 93–95.
- ^ 長沼・井田 2014, pp. 94–95.
- ^ a b c 今村剛 (JAXA宇宙科学研究所). “金星の気候の謎”. 岩波書店「科学」2007年2月号掲載記事からの改変. JAXA. 2019年12月16日閲覧。
- ^ 江守正多 (温暖化リスク評価研究室長) (2010年3月). “ココが知りたい地球温暖化 | Q15 温暖化は暴走する?”. 国立環境研究所 地球環境研究センター. 2019年12月19日閲覧。
- ^ a b c 佐々木貴教. “ハビタブルゾーンとハビタブルプラネット”. Extrasolar Planet's Catalogue. 京都大学. 2019年7月23日閲覧。
- ^ a b c d Kopparapu, Ravi Kumar (2013). “A revised estimate of the occurrence rate of terrestrial planets in the habitable zones around kepler m-dwarfs”. The Astrophysical Journal Letters 767 (1): L8. arXiv:1303.2649. Bibcode: 2013ApJ...767L...8K. doi:10.1088/2041-8205/767/1/L8 .
- ^ a b Zsom, Andras; Seager, Sara; De Wit, Julien (2013). “Towards the Minimum Inner Edge Distance of the Habitable Zone”. The Astrophysical Journal 778 (2): 109. arXiv:1304.3714. Bibcode: 2013ApJ...778..109Z. doi:10.1088/0004-637X/778/2/109.
- ^ Pierrehumbert, Raymond; Gaidos, Eric (2011). “Hydrogen Greenhouse Planets Beyond the Habitable Zone”. The Astrophysical Journal Letters 734 (1): L13. arXiv:1105.0021. Bibcode: 2011ApJ...734L..13P. doi:10.1088/2041-8205/734/1/L13.
- ^ a b c Ramirez, Ramses; Kaltenegger, Lisa (2017). “A Volcanic Hydrogen Habitable Zone”. The Astrophysical Journal Letters 837 (1): L4. arXiv:1702.08618. Bibcode: 2017ApJ...837L...4R. doi:10.3847/2041-8213/aa60c8.
- ^ “Stellar habitable zone calculator”. University of Washington. 2019年7月23日閲覧。
- ^ “遠日点”. 天文学辞典. 公益社団法人日本天文学会. 2020年1月27日閲覧。
- ^ “Venus”. Case Western Reserve University (2006年9月13日). 2012年4月26日時点のオリジナルよりアーカイブ。2019年7月23日閲覧。
- ^ Landis, Geoffrey A. (2003). “Colonization of Venus”. AIP Conf. Proc. (米国物理学協会 (AIP)) 654 (1): 1193–1198. Bibcode: 2003AIPC..654.1193L. doi:10.1063/1.1541418. オリジナルの2012-07-11時点におけるアーカイブ。 .
- ^ Sharp, Tim (2017年10月31日). “Atmosphere of the Moon”. Space.com. TechMediaNetwork. 2019年7月23日閲覧。
- ^ Bolonkin, Alexander A. (2009). Artificial Environments on Mars. Berlin Heidelberg: Springer. pp. 599–625. ISBN 978-3-642-03629-3
- ^ a b Haberle, Robert M.; McKay, Christopher P.; Schaeffer, James; Cabrol, Nathalie A.; Grin, Edmon A.; Zent, Aaron P.; Quinn, Richard (2001). “On the possibility of liquid water on present-day Mars”. Journal of Geophysical Research 106 (E10): 23317. Bibcode: 2001JGR...10623317H. doi:10.1029/2000JE001360. ISSN 0148-0227.
- ^ Mann, Adam (2014年2月18日). “Strange Dark Streaks on Mars Get More and More Mysterious”. Wired. 2019年7月23日閲覧。
- ^ “NASA Finds Possible Signs of Flowing Water on Mars”. voanews.com (2011年8月3日). 2017年6月2日時点のオリジナルよりアーカイブ。2019年7月23日閲覧。
- ^ Kerr, Richard A. (2011年8月4日). “Is Mars Weeping Salty Tears?”. news.sciencemag.org. 2011年8月14日時点のオリジナルよりアーカイブ。2019年7月23日閲覧。
- ^ Webster, Guy (2013年12月10日). “NASA Mars Spacecraft Reveals a More Dynamic Red Planet”. Jet Propulsion Laboratory, NASA. 2019年7月23日閲覧。
- ^ “火星の斜面の暗い筋模様、液体ではなく砂の可能性”. アストロアーツ (2017年11月27日). 2020年1月28日閲覧。 “NASA Jet Propulsion Laboratory発表記事 "Recurring Martian Streaks: Flowing Sand, Not Water?" (2017年11月20日) の翻訳・要約引用”
- ^ Salvador, A.; Massol, H.; Davaille, A.; Marcq, E.; Sarda, P.; Chassefière, E. (2017). “The relative influence of H2 O and CO2 on the primitive surface conditions and evolution of rocky planets”. Journal of Geophysical Research: Planets 122 (7): 1458–1486. Bibcode: 2017JGRE..122.1458S. doi:10.1002/2017JE005286. ISSN 2169-9097.
- ^ Chio, Charles Q. (2010年6月22日). “Flashback: Water on Mars Announced 10 Years Ago”. SPACE.com. 2019年7月23日閲覧。
- ^ “Science@NASA, The Case of the Missing Mars Water” (2001年1月5日). 2009年3月27日時点のオリジナルよりアーカイブ。2019年7月23日閲覧。
- ^ Scully, Jennifer E.C.; Russell, Christopher T.; Yin, An; Jaumann, Ralf; Carey, Elizabeth; Castillo-Rogez, Julie; McSween, Harry Y.; Raymond, Carol A. et al. (2015). “Geomorphological evidence for transient water flow on Vesta”. Earth and Planetary Science Letters 411: 151–163. Bibcode: 2015E&PSL.411..151S. doi:10.1016/j.epsl.2014.12.004. ISSN 0012-821X.
- ^ Raponi, Andrea; De Sanctis, Maria Cristina; Frigeri, Alessandro; Ammannito, Eleonora; Ciarniello, Mauro; Formisano, Michelangelo; Combe, Jean-Philippe; Magni, Gianfranco et al. (2018). “Variations in the amount of water ice on Ceres' surface suggest a seasonal water cycle”. Science Advances 4 (3): eaao3757. Bibcode: 2018SciA....4O3757R. doi:10.1126/sciadv.aao3757. ISSN 2375-2548. PMC 5851659. PMID 29546238 .
- ^ “PIA21471: Landslides on Ceres”. Jet Propulsion Laboratory, NASA (2017年4月19日). 2020年1月28日閲覧。
- ^ “新用語解説 放射強制力” (PDF). 気候変動に関する政府間パネル(IPCC). 日本気象学会 (2009年12月). 2020年1月28日閲覧。
- ^ “放射強制力”. ブリタニカ国際大百科事典 小項目事典. コトバンク. 2020年1月28日閲覧。
- ^ Budyko, M. I. (1969). “The effect of solar radiation variations on the climate of the Earth”. Tellus 21 (5): 611–619. Bibcode: 1969TellA..21..611B. doi:10.1111/j.2153-3490.1969.tb00466.x.
- ^ Sellers, William D. (1969). “A Global Climatic Model Based on the Energy Balance of the Earth-Atmosphere System”. Journal of Applied Meteorology 8 (3): 392–400. Bibcode: 1969JApMe...8..392S. doi:10.1175/1520-0450(1969)008<0392:AGCMBO>2.0.CO;2.
- ^ North, Gerald R. (1975). “Theory of Energy-Balance Climate Models”. Journal of the Atmospheric Sciences 32 (11): 2033–2043. Bibcode: 1975JAtS...32.2033N. doi:10.1175/1520-0469(1975)032<2033:TOEBCM>2.0.CO;2.
- ^ Rasool, I.; De Bergh, C. (1970). “The Runaway Greenhouse and the Accumulation of CO2 in the Venus Atmosphere” (PDF). Nature 226 (5250): 1037–1039. Bibcode: 1970Natur.226.1037R. doi:10.1038/2261037a0. ISSN 0028-0836. PMID 16057644 .
- ^ Hart, M. H. (1979). “Habitable zones about main sequence stars”. Icarus 37 (1): 351–357. Bibcode: 1979Icar...37..351H. doi:10.1016/0019-1035(79)90141-6.
- ^ Spiegel, D. S.; Raymond, S. N.; Dressing, C. D.; Scharf, C. A.; Mitchell, J. L. (2010). “Generalized Milankovitch Cycles and Long-Term Climatic Habitability”. The Astrophysical Journal 721 (2): 1308–1318. arXiv:1002.4877. Bibcode: 2010ApJ...721.1308S. doi:10.1088/0004-637X/721/2/1308.
- ^ Abe, Y.; Abe-Ouchi, A.; Sleep, N. H.; Zahnle, K. J. (2011). “Habitable Zone Limits for Dry Planets”. Astrobiology 11 (5): 443–460. Bibcode: 2011AsBio..11..443A. doi:10.1089/ast.2010.0545. PMID 21707386.
- ^ a b Pierrehumbert, Raymond; Gaidos, Eric (2011). “Hydrogen Greenhouse Planets Beyond the Habitable Zone”. The Astrophysical Journal Letters 734 (1): L13. arXiv:1105.0021. Bibcode: 2011ApJ...734L..13P. doi:10.1088/2041-8205/734/1/L13.
- ^ a b c Vladilo, Giovanni; Murante, Giuseppe; Silva, Laura; Provenzale, Antonello; Ferri, Gaia; Ragazzini, Gregorio (2013). “The habitable zone of Earth-like planets with different levels of atmospheric pressure”. The Astrophysical Journal 767 (1): 65–?. arXiv:1302.4566. Bibcode: 2013ApJ...767...65V. doi:10.1088/0004-637X/767/1/65 .
- ^ Kopparapu, Ravi Kumar; Ramirez, Ramses; Kasting, James F.; Eymet, Vincent; Robinson, Tyler D.; Mahadevan; Terrien, Ryan C.; Domagal-Goldman, Shawn et al. (2013). “Habitable Zones Around Main-Sequence Stars: New Estimates”. The Astrophysical Journal 765: 131. arXiv:1301.6674. Bibcode: 2013ApJ...765..131K. doi:10.1088/0004-637X/765/2/131 .
- ^ Leconte, Jeremy; Forget, Francois; Charnay, Benjamin; Wordsworth, Robin; Pottier, Alizee (2013). “Increased insolation threshold for runaway greenhouse processes on Earth like planets”. Nature 504 (7479): 268–271. arXiv:1312.3337. Bibcode: 2013Natur.504..268L. doi:10.1038/nature12827. PMID 24336285.
- ^ Cuntz, Manfred (2013). “S-Type and P-Type Habitability in Stellar Binary Systems: A Comprehensive Approach. I. Method and Applications”. The Astrophysical Journal 780 (1): 14. arXiv:1303.6645. Bibcode: 2014ApJ...780...14C. doi:10.1088/0004-637X/780/1/14.
- ^ Forget, F.; Pierrehumbert, R. T. (1997). “Warming Early Mars with Carbon Dioxide Clouds That Scatter Infrared Radiation”. Science 278 (5341): 1273–1276. Bibcode: 1997Sci...278.1273F. doi:10.1126/science.278.5341.1273. PMID 9360920.
- ^ Mischna, M.; Kasting, J. F.; Pavlov, A.; Freedman, R. (2000). “Influence of Carbon Dioxide Clouds on Early Martian Climate”. Icarus 145 (2): 546–554. Bibcode: 2000Icar..145..546M. doi:10.1006/icar.2000.6380. PMID 11543507.
- ^ Vu, Linda. "Planets Prefer Safe Neighborhoods" (Press release). Spitzer.caltech.edu. NASA/Caltech. 2019年7月23日閲覧。
- ^ Buccino, Andrea P.; Lemarchand, Guillermo A.; Mauas, Pablo J. D. (2006). “Ultraviolet radiation constraints around the circumstellar habitable zones”. Icarus 183 (2): 491–503. arXiv:astro-ph/0512291. doi:10.1016/j.icarus.2006.03.007.
- ^ a b Barnes, Rory; Heller, René (2013). “Habitable Planets Around White and Brown Dwarfs: The Perils of a Cooling Primary”. Astrobiology 13 (3): 279–291. arXiv:1203.5104. Bibcode: 2013AsBio..13..279B. doi:10.1089/ast.2012.0867. PMC 3612282. PMID 23537137 .
- ^ a b Yang, J.; Cowan, N. B.; Abbot, D. S. (2013). “Stabilizing Cloud Feedback Dramatically Expands the Habitable Zone of Tidally Locked Planets”. The Astrophysical Journal 771 (2): L45. arXiv:1307.0515. Bibcode: 2013ApJ...771L..45Y. doi:10.1088/2041-8205/771/2/L45.
- ^ Agol, Eric (2011). “Transit Surveys for Earths in the Habitable Zones of White Dwarfs”. The Astrophysical Journal Letters 731 (2): 1–5. arXiv:1103.2791. Bibcode: 2011ApJ...731L..31A. doi:10.1088/2041-8205/731/2/L31 .
- ^ Ramirez, Ramses; Kaltenegger, Lisa (2014). “Habitable Zones of Pre-Main-Sequence Stars”. The Astrophysical Journal Letters 797 (2): L25. arXiv:1412.1764. Bibcode: 2014ApJ...797L..25R. doi:10.1088/2041-8205/797/2/L25.
- ^ Carroll, Bradley W.; Ostlie, Dale A. (2007). An Introduction to Modern Astrophysics (2nd ed.)
- ^ Richmond, Michael (2004年11月10日). “Late stages of evolution for low-mass stars”. Rochester Institute of Technology. 2019年7月23日閲覧。
- ^ Guo, J.; Zhang, F.; Chen, X.; Han, Z. (2009). “Probability distribution of terrestrial planets in habitable zones around host stars”. Astrophysics and Space Science 323 (4): 367–373. arXiv:1003.1368. Bibcode: 2009Ap&SS.323..367G. doi:10.1007/s10509-009-0081-z.
- ^ Kasting, J. F.; Ackerman, T. P. (1986). “Climatic Consequences of Very High Carbon Dioxide Levels in the Earth's Early Atmosphere” (PDF). Science 234 (4782): 1383–1385. doi:10.1126/science.11539665. PMID 11539665 .
- ^ a b Franck, S.; von Bloh, W.; Bounama, C.; Steffen, M.; Schönberner, D.; Schellnhuber, H.-J. (2002). "Habitable Zones and the Number of Gaia's Sisters" (PDF). In Montesinos, Benjamin; Giménez, Alvaro; Guinan, Edward F. (eds.). ASP Conference Series. The Evolving Sun and its Influence on Planetary Environments. Astronomical Society of the Pacific. pp. 261–272. Bibcode:2002ASPC..269..261F. ISBN 1-58381-109-5。
- ^ Croswell, Ken (2001年1月27日). “Red, willing and able” (Full reprint). New Scientist. 2019年7月23日閲覧。
- ^ Alekseev, I. Y.; Kozlova, O. V. (2002). “Starspots and active regions on the emission red dwarf star LQ Hydrae”. Astronomy and Astrophysics 396: 203–211. Bibcode: 2002A&A...396..203A. doi:10.1051/0004-6361:20021424.
- ^ a b Alpert, Mark (2005年11月7日). “Red Star Rising”. 2019年7月23日閲覧。
- ^ Research Corporation (2006). “Andrew West: 'Fewer flares, starspots for older dwarf stars'”. EarthSky .
- ^ Cain, Fraser (2007年). “AstronomyCast episode 40: American Astronomical Society Meeting, May 2007”. Universe Today. 2007年9月26日時点のオリジナルよりアーカイブ。2019年7月23日閲覧。
- ^ Ray Villard (2009年7月27日). “Living in a Dying Solar System, Part 1”. Astrobiology. 2019年7月23日閲覧。
- ^ Christensen, Bill (2005年4月1日). “Red Giants and Planets to Live On”. Space.com. TechMediaNetwork 2019年7月23日閲覧。
- ^ a b c Ramirez, Ramses; Kaltenegger, Lisa (2016). “Habitable Zones of Post-Main Sequence Stars”. The Astrophysical Journal 823 (1): 6. arXiv:1605.04924. Bibcode: 2016ApJ...823....6R. doi:10.3847/0004-637X/823/1/6.
- ^ a b Lopez, B.; Schneider, J.; Danchi, W. C. (2005). “Can Life Develop in the Expanded Habitable Zones around Red Giant Stars?”. The Astrophysical Journal 627 (2): 974–985. arXiv:astro-ph/0503520. Bibcode: 2005ApJ...627..974L. doi:10.1086/430416.
- ^ Lorenz, Ralph D.; Lunine, Jonathan I.; McKay, Christopher P. (1997). “Titan under a red giant sun: A new kind of "habitable" moon”. Geophysical Research Letters 24 (22): 2905–2908. Bibcode: 1997GeoRL..24.2905L. doi:10.1029/97GL52843. ISSN 0094-8276. PMID 11542268.
- ^ Voisey, Jon (2011年2月23日). “Plausibility Check – Habitable Planets around Red Giants”. Universe Today 2019年7月23日閲覧。
- ^ Alien Life More Likely on 'Dune' Planets Archived December 2, 2013, at the Wayback Machine., 09/01/11, Charles Q. Choi, Astrobiology Magazine
- ^ Habitable Zone Limits for Dry Planets, Yutaka Abe, Ayako Abe-Ouchi, Norman H. Sleep, and Kevin J. Zahnle. Astrobiology. June 2011, 11(5): 443–460. doi:10.1089/ast.2010.0545
- ^ Drake, Michael J. (2005). “Origin of water in the terrestrial planets”. Meteoritics & Planetary Science 40 (4): 519–527. Bibcode: 2005M&PS...40..519D. doi:10.1111/j.1945-5100.2005.tb00960.x.
- ^ Drake, Michael J.; Humberto, Campins (2005). "Origin of water in the terrestrial planets". Asteroids, Comets, and Meteors (IAU S229). 229th Symposium of the International Astronomical Union. Vol. 1. Búzios, Rio de Janeiro, Brazil: Cambridge University Press. pp. 381–394. Bibcode:2006IAUS..229..381D. doi:10.1017/S1743921305006861. ISBN 978-0-521-85200-5。
- ^ Kuchner, Marc (2003). “Volatile-rich Earth-Mass Planets in the Habitable Zone”. Astrophysical Journal 596 (1): L105–L108. arXiv:astro-ph/0303186. Bibcode: 2003ApJ...596L.105K. doi:10.1086/378397.
- ^ Charbonneau, David; Zachory K. Bert; Jonathan Irwin; Christopher J. Burke; Philip Nutzman; Lars A. Buchhave; Christophe Lovis; Xavier Bonfils et al. (2009). “A super-Earth transiting a nearby low-mass star”. Nature 462 (17 December 2009): 891–894. arXiv:0912.3229. Bibcode: 2009Natur.462..891C. doi:10.1038/nature08679. PMID 20016595 .
- ^ Kuchner, Seager; Hier-Majumder, M.; Militzer, C. A. (2007). “Mass–radius relationships for solid exoplanets”. The Astrophysical Journal 669 (2): 1279–1297. arXiv:0707.2895. Bibcode: 2007ApJ...669.1279S. doi:10.1086/521346 .
- ^ Vastag, Brian (2011年12月5日). “Newest alien planet is just the right temperature for life”. The Washington Post 2019年7月23日閲覧。
- ^ Robinson, Tyler D.; Catling, David C. (2012). “An Analytic Radiative-Convective Model for Planetary Atmospheres”. The Astrophysical Journal 757 (1): 104. arXiv:1209.1833. Bibcode: 2012ApJ...757..104R. doi:10.1088/0004-637X/757/1/104.
- ^ Shizgal, B. D.; Arkos, G. G. (1996). “Nonthermal escape of the atmospheres of Venus, Earth, and Mars”. Reviews of Geophysics 34 (4): 483–505. Bibcode: 1996RvGeo..34..483S. doi:10.1029/96RG02213.
- ^ D. P. Hamilton; J. A. Burns (1992). “Orbital stability zones about asteroids. II – The destabilizing effects of eccentric orbits and of solar radiation” (PDF). Icarus 96 (1): 43–64. Bibcode: 1992Icar...96...43H. doi:10.1016/0019-1035(92)90005-R .
- ^ Becquerel P. (1950). “La suspension de la vie au dessous de 1/20 K absolu par demagnetization adiabatique de l'alun de fer dans le vide les plus eléve” (French). C. R. Acad. Sci. Paris 231: 261–263.
- ^ Horikawa, Daiki D. (2012). Alexander V. Altenbach, Joan M. Bernhard & Joseph Seckbach. ed. Anoxia Evidence for Eukaryote Survival and Paleontological Strategies. (21st ed.). Springer Netherlands. pp. 205–217. ISBN 978-94-007-1895-1
- ^ Kane, Stephen R.; Gelino, Dawn M. (2012). “The Habitable Zone and Extreme Planetary Orbits”. Astrobiology 12 (10): 940–945. arXiv:1205.2429. Bibcode: 2012AsBio..12..940K. doi:10.1089/ast.2011.0798. PMID 23035897.
- ^ Paul Gilster (2015年1月30日). “A Review of the Best Habitable Planet Candidates”. Centauri Dreams, Tau Zero Foundation. 2019年7月23日閲覧。
- ^ Giovanni F. Bignami (2015). The Mystery of the Seven Spheres: How Homo sapiens will Conquer Space. Springer. p. 110. ISBN 978-3-319-17004-6
- ^ Wethington, Nicholos (2008年9月16日). “How Many Stars are in the Milky Way?”. Universe Today 2019年7月23日閲覧。
- ^ a b Torres, Abel Mendez (2013年4月26日). “Ten potentially habitable exoplanets now”. Habitable Exoplanets Catalog. University of Puerto Rico. 2019年7月23日閲覧。
- ^ Borenstein, Seth (2011年2月19日). “Cosmic census finds crowd of planets in our galaxy”. Associated Press. オリジナルの2011年9月27日時点におけるアーカイブ。 2019年7月23日閲覧。
- ^ Choi, Charles Q. (2011年3月21日). “New Estimate for Alien Earths: 2 Billion in Our Galaxy Alone”. Space.com. 2019年7月23日閲覧。
- ^ Catanzarite, J.; Shao, M. (2011). “The Occurrence Rate of Earth Analog Planets Orbiting Sun-Like Stars”. The Astrophysical Journal 738 (2): 151. arXiv:1103.1443. Bibcode: 2011ApJ...738..151C. doi:10.1088/0004-637X/738/2/151.
- ^ Williams, D.; Pollard, D. (2002). “Earth-like worlds on eccentric orbits: excursions beyond the habitable zone”. International Journal of Astrobiology 1 (1): 61–69. Bibcode: 2002IJAsB...1...61W. doi:10.1017/S1473550402001064 .
- ^ “70 Virginis b”. Extrasolar Planet Guide. Extrasolar.net. 2012年6月19日時点のオリジナルよりアーカイブ。2019年7月23日閲覧。
- ^ Williams, D.; Pollard, D. (2002). “Earth-like worlds on eccentric orbits: excursions beyond the habitable zone”. International Journal of Astrobiology 1 (1): 61–69. Bibcode: 2002IJAsB...1...61W. doi:10.1017/S1473550402001064 .
- ^ Sudarsky, David; Burrows, Adam; Hubeny, Ivan (2003). “Theoretical Spectra and Atmospheres of Extrasolar Giant Planets”. The Astrophysical Journal 588 (2): 1121–1148. arXiv:astro-ph/0210216. Bibcode: 2003ApJ...588.1121S. doi:10.1086/374331 .
- ^ Jones, B. W.; Sleep, P. N.; Underwood, D. R. (2006). “Habitability of Known Exoplanetary Systems Based on Measured Stellar Properties”. The Astrophysical Journal 649 (2): 1010–1019. arXiv:astro-ph/0603200. Bibcode: 2006ApJ...649.1010J. doi:10.1086/506557.
- ^ Butler, R. P.; Wright, J. T.; Marcy, G. W.; Fischer, D. A.; Vogt, S. S.; Tinney, C. G.; Jones, H. R. A.; Carter, B. D. et al. (2006). “Catalog of Nearby Exoplanets”. The Astrophysical Journal 646 (1): 505–522. arXiv:astro-ph/0607493. Bibcode: 2006ApJ...646..505B. doi:10.1086/504701.
- ^ Canup, R. M.; Ward, W. R. (2006). “A common mass scaling for satellite systems of gaseous planets”. Nature 441 (7095): 834–839. Bibcode: 2006Natur.441..834C. doi:10.1038/nature04860. PMID 16778883.
- ^ Barnes, J. W.; O'Brien, D. P. (2002). “Stability of Satellites around Close‐in Extrasolar Giant Planets”. The Astrophysical Journal 575 (2): 1087–1093. arXiv:astro-ph/0205035. Bibcode: 2002ApJ...575.1087B. doi:10.1086/341477.
- ^ Lovis, C.; Mayor, M.; Pepe, F.; Alibert, Y.; Benz, W.; Bouchy, F.; Correia, A. C.; Laskar, J. et al. (2006). “An extrasolar planetary system with three Neptune-mass planets”. Nature 441 (7091): 305–309. arXiv:astro-ph/0703024. Bibcode: 2006Natur.441..305L. doi:10.1038/nature04828. PMID 16710412 .
- ^ a b “Astronomers Discover Record Fifth Planet Around Nearby Star 55 Cancri”. Sciencedaily.com (2007年11月6日). 2019年7月23日閲覧。
- ^ Fischer, Debra A.; Marcy, Geoffrey W.; Butler, R. Paul; Vogt, Steven S.; Laughlin, Greg; Henry, Gregory W.; Abouav, David; Peek, Kathryn M. G. et al. (2008). “Five Planets Orbiting 55 Cancri”. The Astrophysical Journal 675 (1): 790–801. arXiv:0712.3917. Bibcode: 2008ApJ...675..790F. doi:10.1086/525512 .
- ^ Ian Sample, science correspondent (2007年11月7日). “Could this be Earth's near twin? Introducing planet 55 Cancri f”. The Guardian 2019年7月23日閲覧。
- ^ Than, Ker (2007年2月24日). “Planet Hunters Edge Closer to Their Holy Grail”. Space.com 2019年7月23日閲覧。
- ^ Rpbertspm, R.; Mahadevan, S.; Endl, M; Roy, A. (2014). “Stellar activity masquerading as planets in the habitable zone of the M dwarf Glise 581”. Science 345 (6195): 440-444. doi:10.1126/science.1253253.
- ^ Robertson, Paul; Mahadevan, Suvrath; Endl, Michael; Roy, Arpita (2014). “Stellar activity masquerading as planets in the habitable zone of the M dwarf Gliese 581”. Science 345 (6195): 440–444. arXiv:1407.1049. Bibcode: 2014Sci...345..440R. doi:10.1126/science.1253253. PMID 24993348.
- ^ “Researchers find potentially habitable planet” (French). maxisciences.com (2011年8月30日). 2019年7月23日閲覧。
- ^ “Kepler 22-b: Earth-like planet confirmed”. BBC. (2011年12月5日) 2019年7月23日閲覧。
- ^ Scharf, Caleb A. (2011年12月8日). “You Can't Always Tell an Exoplanet by Its Size”. 2019年7月23日閲覧。: "If it [Kepler-22b] had a similar composition to Earth, then we're looking at a world in excess of about 40 Earth masses".
- ^ Anglada-Escude, Guillem; Arriagada, Pamela; Vogt, Steven; Rivera, Eugenio J.; Butler, R. Paul; Crane, Jeffrey D.; Shectman, Stephen A.; Thompson, Ian B. et al. (2012). “A planetary system around the nearby M dwarf GJ 667C with at least one super-Earth in its habitable zone”. The Astrophysical Journal 751 (1): L16. arXiv:1202.0446. Bibcode: 2012ApJ...751L..16A. doi:10.1088/2041-8205/751/1/L16.
- ^ “Results for HIP 19394”. SIMBAD Astronomical Database. CDS. 2019年7月23日閲覧。
- ^ Méndez, Abel (2012年8月29日). “A Hot Potential Habitable Exoplanet around Gliese 163”. Planetary Habitability Laboratory. University of Puerto Rico at Arecibo. 2019年7月23日閲覧。
- ^ Nola Taylor Redd (2012年9月20日). “Newfound Alien Planet a Top Contender to Host Life”. Space.com. 2019年7月23日閲覧。
- ^ “A Hot Potential Habitable Exoplanet around Gliese 163”. Spacedaily.com. 2019年7月23日閲覧。
- ^ Tuomi, Mikko; Anglada-Escude, Guillem; Gerlach, Enrico; Jones, Hugh R. R.; Reiners, Ansgar; Rivera, Eugenio J.; Vogt, Steven S.; Butler, Paul (2012). “Habitable-zone super-Earth candidate in a six-planet system around the K2.5V star HD 40307”. Astronomy and Astrophysics 549: A48. arXiv:1211.1617. Bibcode: 2013A&A...549A..48T. doi:10.1051/0004-6361/201220268.
- ^ Aron, Jacob (2012年12月19日). “Nearby Tau Ceti may host two planets suited to life”. New Scientist. Reed Business Information. 2019年7月23日閲覧。
- ^ Tuomi, M.; Jones, H. R. A.; Jenkins, J. S.; Tinney, C. G.; Butler, R. P.; Vogt, S. S.; Barnes, J. R.; Wittenmyer, R. A. et al. (2013). “Signals embedded in the radial velocity noise”. Astronomy and Astrophysics 551: A79. arXiv:1212.4277. Bibcode: 2013A&A...551A..79T. doi:10.1051/0004-6361/201220509.
- ^ Torres, Abel Mendez (2013年5月1日). “The Habitable Exoplanets Catalog”. Habitable Exoplanets Catalog. University of Puerto Rico. 2019年7月23日閲覧。
- ^ Lauren M. Weiss; Geoffrey W. Marcy (2014). “The mass-radius relation for 65 exoplanets smaller than 4 Earth radii”. The Astrophysical Journal Letters 783 (1): 7. arXiv:1312.0936. Bibcode: 2014ApJ...783L...6W. doi:10.1088/2041-8205/783/1/L6 .
- ^ “Solar Variability and Terrestrial Climate”. NASA Science (2013年1月8日). 2019年7月23日閲覧。
- ^ “Stellar Luminosity Calculator”. University of Nebraska-Lincoln astronomy education group. 2019年7月23日閲覧。
- ^ Council, National Research (18 September 2012). The Effects of Solar Variability on Earth's Climate: A Workshop Report. doi:10.17226/13519. ISBN 978-0-309-26564-5
- ^ Most of Earth's twins aren't identical, or even close!, By Ethan. June 5, 2013.
- ^ “Are there oceans on other planets?”. National Oceanic and Atmospheric Administration (2017年7月6日). 2019年7月23日閲覧。
- ^ a b Johnson, Michele (2013年4月18日). “NASA's Kepler Discovers Its Smallest 'Habitable Zone' Planets to Date”. NASA. 2019年7月23日閲覧。
- ^ a b Overbye, Dennis (2013年4月18日). “Two Promising Places to Live, 1,200 Light-Years from Earth”. The New York Times 2019年7月23日閲覧。
- ^ Moskowitz, Clara (2013年1月9日). “Most Earth-Like Alien Planet Possibly Found”. Space.com. 2019年7月23日閲覧。
- ^ Barclay, Thomas; Burke, Christopher J.; Howell, Steve B.; Rowe, Jason F.; Huber, Daniel; Isaacson, Howard; Jenkins, Jon M.; Kolbl, Rea et al. (2013). “A Super-Earth-Sized Planet Orbiting in or Near the Habitable Zone Around a Sun-Like Star”. The Astrophysical Journal 768 (2): 101. arXiv:1304.4941. Bibcode: 2013ApJ...768..101B. doi:10.1088/0004-637X/768/2/101.
- ^ Stephen R. Kane; Thomas Barclay; Dawn M. Gelino. “A Potential Super-Venus in the Kepler-69 System”. The Astrophysical Journal Letters 770 (2): L20. arXiv:1305.2933. Bibcode: 2013ApJ...770L..20K. doi:10.1088/2041-8205/770/2/L20.
- ^ Borucki, William J. et al. (2013). “Kepler-62: A Five-Planet System with Planets of 1.4 and 1.6 Earth Radii in the Habitable Zone”. Science Express 340 (6132): 587–590. arXiv:1304.7387. Bibcode: 2013Sci...340..587B. doi:10.1126/science.1234702. PMID 23599262 .
- ^ Chang, Kenneth (2014年4月7日). “Scientists Find an 'Earth Twin,' or Maybe a Cousin”. The New York Times 2019年7月23日閲覧。
- ^ Chang, Alicia (2014年4月17日). “Astronomers spot most Earth-like planet yet”. AP News 2019年7月23日閲覧。
- ^ Morelle, Rebecca (2014年4月17日). “'Most Earth-like planet yet' spotted by Kepler”. BBC News 2019年7月23日閲覧。
- ^ Wall, Mike (2014年6月3日). “Found! Oldest Known Alien Planet That Might Support Life”. Space.com. 2019年7月23日閲覧。
- ^ Robertson, Paul; Roy, Arpita; Mahadevan, Suvrath (2015). “Stellar activity mimics a habitable-zone planet around Kapteyn's star”. The Astrophysical Journal 805 (2): L22. arXiv:1505.02778. Bibcode: 2015ApJ...805L..22R. doi:10.1088/2041-8205/805/2/L22. ISSN 2041-8213.
- ^ a b c Clavin, Whitney (2015年1月6日). “NASA's Kepler Marks 1,000th Exoplanet Discovery, Uncovers More Small Worlds in Habitable Zones”. NASA. 2019年7月23日閲覧。
- ^ Jensen, Mari N. (2015年1月16日). “Three nearly Earth-size planets found orbiting nearby star: One in 'Goldilocks' zone”. Science Daily 2019年7月23日閲覧。
- ^ Jenkins, Jon M.; Twicken, Joseph D.; Batalha, Natalie M.; Caldwell, Douglas A.; Cochran, William D.; Endl, Michael; Latham, David W.; Esquerdo, Gilbert A. et al. (2015). “Discovery and Validation of Kepler-452b: A 1.6 R⨁ Super Earth Exoplanet in the Habitable Zone of a G2 Star”. The Astronomical Journal 150 (2): 56. arXiv:1507.06723. Bibcode: 2015AJ....150...56J. doi:10.1088/0004-6256/150/2/56. ISSN 1538-3881 .
- ^ “NASA telescope discovers Earth-like planet in star's habitable zone”. BNO News (2015-0). 2019年7月23日閲覧。
- ^ “NASA’s Kepler Mission Discovers Bigger, Older Cousin to Earth”. NASA (2015年7月23日). 2018-0-23閲覧。
- ^ Dressing, Courtney D.; Vanderburg, Andrew; Schlieder, Joshua E.; Crossfield, Ian J. M.; Knutson, Heather A.; Newton, Elisabeth R.; Ciardi, David R.; Fulton, Benjamin J. et al. (2017). “Characterizing K2 Candidate Planetary Systems Orbiting Low-mass Stars. II. Planetary Systems Observed During Campaigns 1–7” (PDF). The Astronomical Journal 154 (5): 207. arXiv:1703.07416. Bibcode: 2017AJ....154..207D. doi:10.3847/1538-3881/aa89f2. ISSN 1538-3881 .
- ^ Gillon, Michaël; Triaud, Amaury H. M. J. et al. (2017). “Seven temperate terrestrial planets around the nearby ultracool dwarf star TRAPPIST-1” (PDF). Nature 542 (7642): 456-460. doi:10.1038/nature21360. ISSN 0028-0836 .
- ^ Dittmann, Jason A.; Irwin, Jonathan M.; Charbonneau, David; Bonfils, Xavier; Astudillo-Defru, Nicola; Haywood, Raphaëlle D.; Berta-Thompson, Zachory K.; Newton, Elisabeth R. et al. (2017). “A temperate rocky super-Earth transiting a nearby cool star”. Nature 544 (7650): 333–336. arXiv:1704.05556. Bibcode: 2017Natur.544..333D. doi:10.1038/nature22055. PMID 28426003.
- ^ “Astronomers are beaming techno into space for aliens to decode”. Wired UK (2017年11月16日). 2019年7月23日閲覧。
- ^ “In Earth's Backyard: Newfound Alien Planet May be Good Bet for Life”. Space.com (2017年11月15日). 2019年7月23日閲覧。
- ^ “K2-155 d”. Exoplanet Exploration (2018年). 2019年7月23日閲覧。
- ^ Mack, Eric (2018年3月13日). “A super-Earth around a red star could be wet and wild”. CNET. 2019年7月23日閲覧。
- ^ Whitwam, Ryan (2018年3月14日). “Kepler Spots Potentially Habitable Super-Earth Orbiting Nearby Star”. ExtremeTech. 2019年7月23日閲覧。
- ^ Zechmeister, M.; Dreizler, S.; Ribas, I.; Reiners, A.; Caballero, J. A. (2019). “The CARMENES search for exoplanets around M dwarfs. Two temperate Earth-mass planet candidates around Teegarden's Star”. Astronomy and Astrophysics. arXiv:1906.07196. Bibcode: 2019arXiv190607196Z. doi:10.1051/0004-6361/201935460. ISSN 0004-6361.
- ^ Torres, Abel (2012年6月12日). “Liquid Water in the Solar System”. 2019年7月23日閲覧。
- ^ Munro, Margaret (2013年). “Miners deep underground in northern Ontario find the oldest water ever known”. National Post. 2019年7月23日閲覧。
- ^ Davies, Paul (2013年). “The Origin of Life II: How did it begin?” (PDF). 2019年7月23日閲覧。[リンク切れ]
- ^ Taylor, Geoffrey (1996年). “Life Underground” (PDF). Planetary Science Research Discoveries. 2019年7月23日閲覧。
- ^ Doyle, Alister (2013年3月4日). “Deep underground, worms and "zombie microbes" rule”. Reuters 2019年7月23日閲覧。
- ^ Nicholson, W. L.; Moeller, R.; Horneck, G.; PROTECT Team (2012). “Transcriptomic Responses of Germinating Bacillus subtilis Spores Exposed to 1.5 Years of Space and Simulated Martian Conditions on the EXPOSE-E Experiment PROTECT”. Astrobiology 12 (5): 469–486. Bibcode: 2012AsBio..12..469N. doi:10.1089/ast.2011.0748. PMID 22680693.
- ^ Brownlee, Donald; Ward, Peter (2004). Rare Earth: Why Complex Life Is Uncommon in the Universe. New York: Copernicus. ISBN 978-0-387-95289-5
- ^ Decker, Heinz; Holde, Kensal E. (2011). “Oxygen and the Exploration of the Universe”. Oxygen and the Evolution of Life. pp. 157–168. doi:10.1007/978-3-642-13179-0_9. ISBN 978-3-642-13178-3
- ^ a b Stewart, Ian; Cohen, Jack (2002). Evolving the Alien. Ebury Press. ISBN 978-0-09-187927-3
- ^ Goldsmith, Donald; Owen, Tobias (1992). The Search for Life in the Universe (2nd ed.). Addison-Wesley. p. 247. ISBN 978-0-201-56949-0
- ^ Vaclav Smil (2003). The Earth's Biosphere: Evolution, Dynamics, and Change. MIT Press. p. 166. ISBN 978-0-262-69298-4
- ^ Reynolds, R. T.; McKay, C. P.; Kasting, J. F. (1987). “Europa, Tidally Heated Oceans, and Habitable Zones Around Giant Planets”. Advances in Space Research 7 (5): 125–132. Bibcode: 1987AdSpR...7..125R. doi:10.1016/0273-1177(87)90364-4 .
- ^ Guidetti, R.; Jönsson, K. I. (2002). “Long-term anhydrobiotic survival in semi-terrestrial micrometazoans”. Journal of Zoology 257 (2): 181–187. doi:10.1017/S095283690200078X.
- ^ Baldwin, Emily (2012年4月26日). “Lichen survives harsh Mars environment”. Skymania New. 2019年7月23日閲覧。
- ^ de Vera, J. P. (2012年4月26日). “The adaptation potential of extremophiles to Martian surface conditions and its implication for the habitability of Mars” (PDF). European Geosciences Union. 2012年5月4日時点のオリジナルよりアーカイブ。2019年7月23日閲覧。
- ^ a b Onofri, Silvano; de Vera, Jean-Pierre; Zucconi, Laura; Selbmann, Laura; Scalzi, Giuliano; Venkateswaran, Kasthuri J.; Rabbow, Elke; de la Torre, Rosa et al. (2015). “Survival of Antarctic Cryptoendolithic Fungi in Simulated Martian Conditions On Board the International Space Station”. Astrobiology 15 (12): 1052–1059. Bibcode: 2015AsBio..15.1052O. doi:10.1089/ast.2015.1324. ISSN 1531-1074. PMID 26684504.
- ^ Isler, K.; van Schaik, C. P (2006). “Metabolic costs of brain size evolution”. Biology Letters 2 (4): 557–560. doi:10.1098/rsbl.2006.0538. ISSN 1744-9561. PMC 1834002. PMID 17148287 .
- ^ Palca, Joe. “'Goldilocks' Planet's Temperature Just Right For Life”. 2010-09-29 (NPR) 2019年7月23日閲覧。
- ^ “Project Cyclops: A design study of a system for detecting extraterrestrial intelligent life”. NASA (1971年). 2019年7月23日閲覧。
- ^ Joseph A. Angelo (2007). Life in the Universe. Infobase Publishing. p. 163. ISBN 978-1-4381-0892-6
- ^ Turnbull, Margaret C.; Tarter, Jill C. (2003). “Target Selection for SETI. I. A Catalog of Nearby Habitable Stellar Systems”. The Astrophysical Journal Supplement Series 145 (1): 181–198. arXiv:astro-ph/0210675. Bibcode: 2003ApJS..145..181T. doi:10.1086/345779.
- ^ Siemion, Andrew P. V.; Demorest, Paul; Korpela, Eric; Maddalena, Ron J.; Werthimer, Dan; Cobb, Jeff; Howard, Andrew W.; Langston, Glen et al. (2013). “A 1.1 to 1.9 GHz SETI Survey of the Kepler Field: I. A Search for Narrow-band Emission from Select Targets”. The Astrophysical Journal 767 (1): 94. arXiv:1302.0845. Bibcode: 2013ApJ...767...94S. doi:10.1088/0004-637X/767/1/94.
- ^ Zaitsev, A. L. (2004). "Transmission and reasonable signal searches in the Universe". Horizons of the Universe Передача и поиски разумных сигналов во Вселенной. Plenary presentation at the National Astronomical Conference WAC-2004 "Horizons of the Universe", Moscow, Moscow State University, June 7, 2004 (Russian). Moscow.
- ^ Grinspoon, David (2007年12月12日). “Who Speaks for Earth?”. Seedmagazine.com. 2019年7月23日閲覧。
- ^ P. C. Gregory; D. A. Fischer (2010). “A Bayesian periodogram finds evidence for three planets in 47 Ursae Majoris”. Monthly Notices of the Royal Astronomical Society 403 (2): 731–747. arXiv:1003.5549. Bibcode: 2010MNRAS.403..731G. doi:10.1111/j.1365-2966.2009.16233.x.
- ^ B. Jones; Underwood, David R.; Sleep, P. Nick (2005). “Prospects for Habitable "Earths" in Known Exoplanetary Systems”. The Astrophysical Journal 622 (2): 1091–1101. arXiv:astro-ph/0503178. Bibcode: 2005ApJ...622.1091J. doi:10.1086/428108.
- ^ Moore, Matthew (2008年10月9日). “Messages from Earth sent to distant planet by Bebo”. London: .telegraph.co.uk. オリジナルの2008年10月11日時点におけるアーカイブ。 2019年7月23日閲覧。
- ^ Prantzos, Nikos (2006). “On the "Galactic Habitable Zone"”. Space Science Reviews 135 (1–4): 313–322. arXiv:astro-ph/0612316. Bibcode: 2008SSRv..135..313P. doi:10.1007/s11214-007-9236-9.
- ^ Lineweaver, C. H.; Fenner, Y.; Gibson, B. K. (2004). “The Galactic Habitable Zone and the Age Distribution of Complex Life in the Milky Way”. Science 303 (5654): 59–62. arXiv:astro-ph/0401024. Bibcode: 2004Sci...303...59L. doi:10.1126/science.1092322. PMID 14704421.
- ^ E. Spitoni; F. Matteucci; A. Sozzetti (2014). “The galactic habitable zone of the Milky Way and M31 from chemical evolution models with gas radial flows”. Monthly Notices of the Royal Astronomical Society 440 (3): 2588-2598. arXiv:1403.2268. Bibcode: 2014MNRAS.440.2588S. doi:10.1093/mnras/stu484 .
引用文献[編集]
- 長沼毅、井田茂『地球外生命 われわれは孤独か』岩波書店〈岩波新書 (新赤版) 1469〉、2014年1月21日。ISBN 9784004314691 。
外部リンク[編集]
- 日経サイエンス 2002年3月号 過酷な宇宙で生き残れる場所は
- “Circumstellar Habitable Zone Simulator”. Astronomy Education at the University of Nebraska-Lincoln. 2019年7月23日閲覧。
- “The Habitable Zone Gallery”. 2019年7月23日閲覧。
- “Stars and Habitable Planets”. SolStation. 2011年6月28日時点のオリジナルよりアーカイブ。2019年7月23日閲覧。
- Interstellar Real Estate: Location, Location, Location – Defining the Habitable Zone
- “Exoplanets in relation to host star's current habitable zone”. www.planetarybiology.com. 2019年7月23日閲覧。
- “exoExplorer: a free Windows application for visualizing exoplanet environments in 3D”. www.planetarybiology.com. 2019年7月23日閲覧。
- Shiga, David (2009年11月19日). “Why the universe may be teeming with aliens”. 2019年7月23日閲覧。
- “The New Worlds Observer: a mission for high-resolution spectroscopy of extra-solar terrestrial planets” (PDF). New Worlds. 2019年7月23日閲覧。
- Cockell, Charles S.; Herbst, Tom; Léger, Alain; Absil, O.; Beichman, Charles; Benz, Willy; Brack, Andre; Chazelas, Bruno et al. (2009). “Darwin – an experimental astronomy mission to search for extrasolar planets” (PDF). Experimental Astronomy 23 (1): 435–461. Bibcode: 2009ExA....23..435C. doi:10.1007/s10686-008-9121-x .
- Atkinson, Nancy (2009年3月19日). “JWST Will Provide Capability to Search for Biomarkers on Earth-like Worlds”. Universe Today. 2019年7月23日閲覧。
{{DEFAULTSORT:はひたふるそおん}} [[Category:宇宙科学]] [[Category:天文学に関する記事]]